7.2 Crystallization of Magma
The minerals that make up igneous rocks crystallize (solidify, freeze) at a range of different temperatures. This explains why cooling magma can have some crystals within it and yet remain predominantly liquid. The sequence in which minerals crystallize from a magma as it cools is known as Bowen’s reaction series (Figure 7.6).
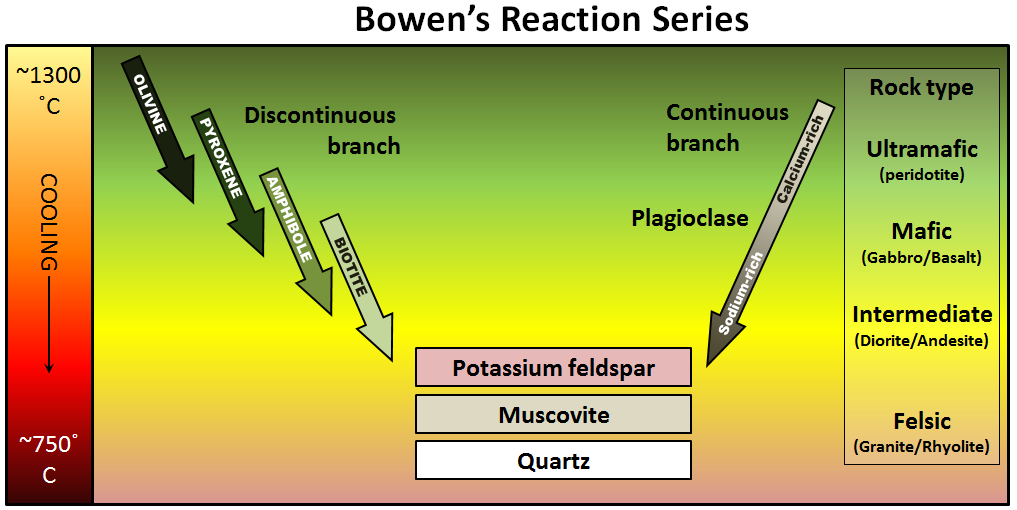
How Did We Get Bowen’s Reaction Series?
Understanding how the reaction series was derived is key to understanding what it means.
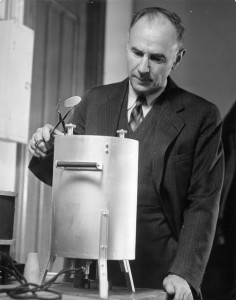
Norman Levi Bowen (Figure 7.7) was born in Kingston Ontario. He studied geology at Queen’s University and then at Massachusetts Institute of Technology. In 1912 he joined the Carnegie Institution in Washington, D.C., where he carried out ground-breaking experiments into how magma cools.
Working mostly with mafic magmas (magmas rich in iron and magnesium), he determined the order of crystallization of minerals as the temperature drops. First, he melted the rock completely in a specially made kiln. Then he allowed it to cool slowly to a specific temperature before quenching (cooling it quickly) so that no new minerals could form. The rocks that formed were studied under the microscope and analyzed chemically. This was done over and over, each time allowing the magma to cool to a lower temperature before quenching.
The result of these experiments was the reaction series which, even a century later, is still an important basis for our understanding of igneous rocks.
Discontinuous and Continuous Series
Bowen’s reaction series (Figure 7.6) has two pathways for minerals to form as magma cools: on the left is the discontinuous series. This refers to the fact that one mineral is transformed into a different mineral through chemical reactions. On the right is the continuous series, where plagioclase feldspar goes from being rich in calcium to being rich in sodium.
Continuous Series
At about the point where pyroxene begins to crystallize, plagioclase feldspar also begins to crystallize. At that temperature, the plagioclase is calcium-rich (toward the anorthite end-member). As the temperature drops, and providing that there is sodium left in the magma, the plagioclase that forms is a more sodium-rich variety (toward the albite end-member). The series is continuous because the mineral is always plagioclase feldspar, but the series involves a transition from calcium-rich to sodium-rich.
When cooling happens relatively quickly, instead of getting crystals which are of uniform composition, individual plagioclase crystals can be zoned from calcium-rich in the centre to more sodium-rich around the outside (Figure 7.8). This occurs because calcium-rich early-forming plagioclase crystals become coated with progressively more sodium-rich plagioclase as the magma cools.
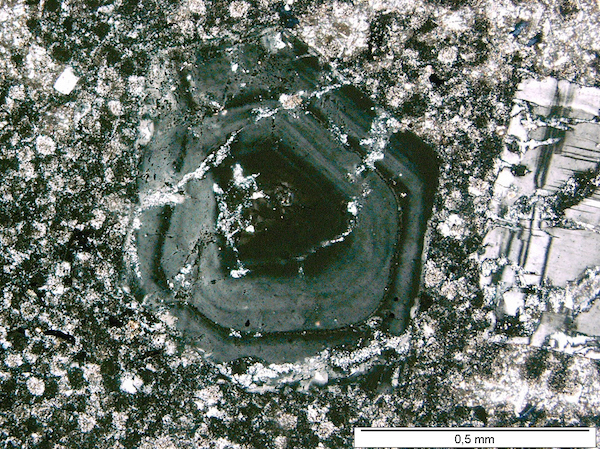
Discontinuous Series
Olivine begins to form at just below 1300°C, but as the temperature drops, olivine becomes unstable. The early-forming olivine crystals react with silica in the remaining liquid and are converted into pyroxene, something like this:
As long as there is silica remaining and the rate of cooling is slow, this process continues down the discontinuous branch: olivine reacts to form pyroxene, and the pyroxene reacts to form amphibole. Under the right conditions amphibole will form to biotite. Finally, if the magma is quite silica-rich to begin with, there will still be some left at around 750 °C to 800 °C, and from this last magma, potassium feldspar, quartz, and maybe muscovite mica will form.
Notice that the sequence of minerals that form goes from isolated tetrahedra (olivine) toward increasingly complex arrangements of silica tetrahedra. Pyroxene consists of single chains, amphibole has double chains, mica has sheets of tetrahedra, and potassium feldspar and quartz at the bottom of the series have tetrahedra connected to each other in three dimensions.
If the magma cools enough, the first minerals to form will be completely used up in later chemical reactions. This is why igneous rocks do not normally have both olivine (at the top of the series) and quartz (at the bottom). Exceptions can occur when rocks that crystallized early in the series come into contact with magmas representing compositions later in the series, such as with the dark green olivine-rich xenoliths included within the quartz- and feldspar-rich rock in Figure 7.9. The dark line around the xenoliths is amphibole, which formed as the olivine reacted with the melt. In some of the smaller xenoliths within this boulder, the olivine has been completely transformed into amphibole.

Magma Composition: Mafic, Intermediate, and Felsic
The composition of the original magma determines how far the reaction process can continue before all of the magma is used up. In other words, it determines which minerals will form. Magma compositions are reported in terms of the fraction of mass of oxides (e.g., Al2O3 rather than just Al; Figure 7.10). On average, mafic[1] magma (Figure 7.10, left) is approximately half SiO2 by mass, and more than 25% iron, magnesium, and calcium oxides by mass. Average felsic[2] magmas (Figure 7.10, right) are closer to 75% SiO2 by mass, and have approximately 5% iron, magnesium, and calcium oxides. Sodium and potassium oxides account for approximately 10% of felsic magmas by mass, but only 5% of mafic magmas. Magmas that fall between mafic and felsic magmas have an intermediate composition (Figure 7.10, centre).

Exercise: Mafic, Intermediate, or Felsic?
The proportions of the main chemical components of felsic, intermediate, and mafic magmas are listed in the table below. (The values are similar to those shown in Figure 7.10).
Oxide | Felsic Magma | Intermediate Magma | Mafic Magma |
SiO2 | 65-75% | 55-65% | 45-55% |
Al2O3 | 12-16% | 14-18% | 14-18% |
FeO | 2-4% | 4-8% | 8-12% |
CaO | 1-4% | 4-7% | 7-11% |
MgO | 0-3% | 2-6% | 5-9% |
Na2O | 2-6% | 3-7% | 1-3% |
K2O | 3-5% | 2-4% | 0.5-3% |
Chemical composition by mass for four rock samples are shown in the following table. Compare these with those in the table above to determine whether each of these samples is felsic, intermediate, or mafic.
SiO2 | Al2O3 | FeO | CaO | MgO | Na2O | K2O | Type? |
55% | 17% | 5% | 6% | 3% | 4% | 3% | |
74% | 14% | 3% | 3% | 0.5% | 5% | 4% | |
47% | 14% | 8% | 10% | 8% | 1% | 2% | |
65% | 14% | 4% | 5% | 4% | 3% | 3% |
What Determines the Composition of Magma?
Why Is There No Ultramafic Magma Anymore?
Partial Melting Makes Magma That Is Richer in Silica
In partial melting, some components of a mixture melt before others do. In the case of mafic magma, it is produced when ultramafic rocks undergo partial melting. In general, silicate minerals with more silica will melt before those with less silica. This means the partial melt will have more silica than the rock as a whole.
Fractional Crystallization Also Makes Magma Richer In Silica
A number of processes that take place within a magma chamber can affect the types of rocks that form once magma cools and crystallizes. If the magma has a low viscosity— which is likely if the magma is mafic—the crystals that form early, such as olivine (Figure 7.11a), may slowly settle toward the bottom of the magma chamber (Figure 7.11b). This process is called fractional crystallization.
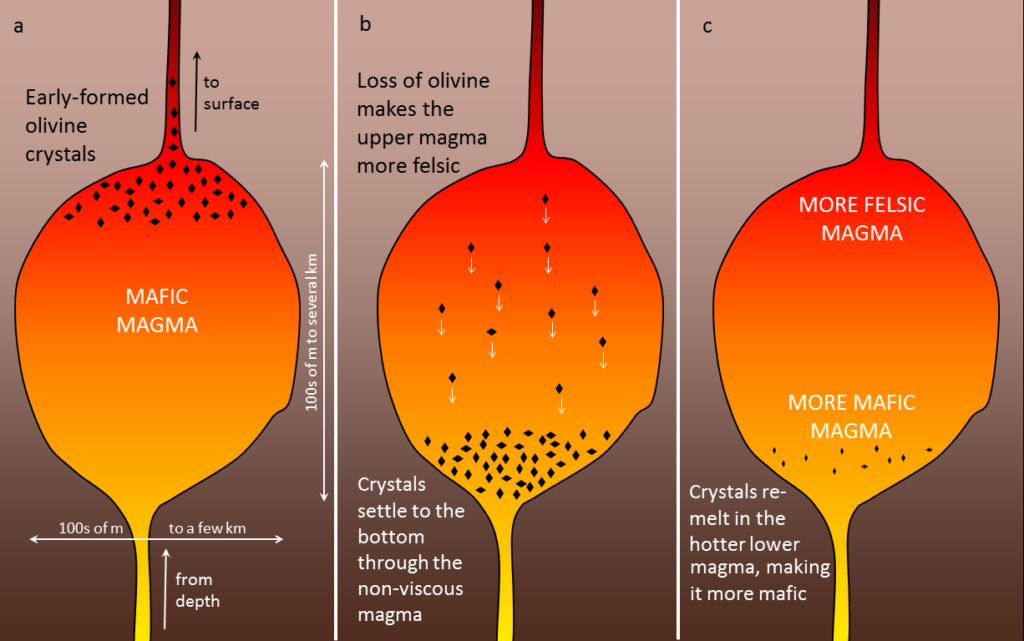
The formation of olivine removes iron- and magnesium-rich components, leaving the overall composition of the magma near the top of the magma chamber more felsic. The crystals that settle might either form an olivine-rich layer near the bottom of the magma chamber. Or, because the lower part of the magma chamber is likely to be hotter than the upper part, the crystals might remelt. If remelting happens, crystal settling will make the magma at the bottom of the chamber more mafic than it was to begin with (Figure 7.11c).
Magma Composition Also Changes When Other Rocks Are Melted And Mixed In
Magma chambers aren’t isolated from their surroundings. If the rock in which the magma chamber is located (called the country rock) is more felsic than the magma, the country rock may also melt, adding to the magma already in the magma chamber (Figure 7.12). Sometimes magma carries fragments of unmelted rock, called xenoliths, within it. Melting of xenoliths can also alter the composition of magma, as can re-melting of crystals that have settled out of the magma.

- "Mafic" combines the words MAgnesium and FerrIC (containing iron). ↵
- "Felsic" combines the words FELdspar and SIliCa. ↵
- The komatiites of the Song Da zone in northwestern Vietnam are 270 million years old, and those on Gorgona Island, Columbia are 89 million years old. Exactly how they formed is still a bit of a mystery. See Table 1 of arXiv:physics/0512118v2 [physics.geo-ph] for a compilation of komatiite ages with references. ↵