Chapter 5. Metamorphic Rocks
Overview of Metamorphic Rocks
Adapted by Lyndsay R. Hauber & Joyce M. McBeth (2018) University of Saskatchewan from Deline B, Harris R & Tefend K. (2015) “Laboratory Manual for Introductory Geology”. First Edition. Chapter 11 “Metamorphic Rocks” by Karen Tefend, CC BY-SA 4.0. View source. Last edited: 28 Dec 2019
5.2 AGENTS OF METAMORPHISM
5.2.1 Pressure
All rocks beneath the surface of the earth experience an increase in pressure due to the weight of the overlying sediment and rock layers, and with increasing depth there is a corresponding increase in pressure. This increased pressure does not necessarily cause a rock to become metamorphic, because this particular pressure is typically equal in all directions and is known as lithostatic pressure. Lithostatic pressure on rocks below the earth’s surface may have a change in overall rock volume, but will not cause a change in the shape. An example of decreasing volume due to lithostatic pressure would be a closer packing of clasts and reduction of pore space within a clastic sedimentary rock. Differential pressure occurs when the pressure on a rock is unequal, and it can result in a significant change in the appearance of a rock. Figure 5.1 demonstrates how a mineral can change shape due to differential pressure, in this case with the greatest pressures from the top and bottom. Two initially rounded mineral grains (e.g. Figure 5.1A) within a sedimentary rock are experiencing the greatest amount of pressure at the contact between the grains (see red arrows in the figure), and the bonds linking the atoms in this grain will break. The atoms will migrate into the area of lesser pressure and reform a bond with other atoms in the mineral grain (e.g. Figure 5.1B). As a result, the grains have a flattened shape that is perpendicular to the direction of greatest pressure (e.g. Figure 5.1C).

Source: Karen Tefend (2015) CC BY-SA 3.0 view source
Figure 5.1 only shows the deformation of two grains; imagine that this is happening to all of the grains in the sedimentary rock, or to all of the phenocrysts (crystals) in an igneous rock. In that case, you will end up with the entire rock having minerals aligned in a certain direction, all by the breaking of bonds between atoms in a mineral, and reforming (recrystallizing) in the lower pressure areas among the grains or crystals in the rock. The end result is a rock with a metamorphic pattern called a foliation. Metamorphic foliations are the patterns seen in a rock that has experienced differential pressure; these foliations may be fairly flat, or have a wavy appearance possibly due to more than one direction of greatest pressure. Some rocks may also develop what is called a lineation, which can be formed by an elongation of minerals that form a linear feature through the rock. To understand the difference between a foliation and a lineation, let us use some food analogies: a stack of pancakes demonstrates a foliation in your breakfast food, with each pancake layer representing flattened minerals. If you look at the top of the pancakes, you will not see a pattern, but if you view the stack of pancakes from the side, or cut through the stack with your knife in any orientation other than parallel to the pancake layers, you will see the layering or foliation. However, if within that pancake stack, there existed a slice of bacon (yum), the bacon would be the lineation in your breakfast “rock”, and you may or may not see it when you cut through the pancake stack. We will discuss foliations, and the different types of foliations, in a later section of this chapter.
5.2.2 Temperature
Probably the most common cause of metamorphism is a change in temperature. Often times metamorphism involves both an increase in temperature along with the pressure changes as described in the above section. Higher temperatures are often associated with metamorphism due to chemically reactive fluids. The broad classification for metamorphism into low, medium and high grades of metamorphic change exists mainly due to temperature conditions.
Higher temperatures increase the vibrational energy between the bonds linking atoms in the mineral structure, making it easier for bonds to be broken in order for the recrystallization of the minerals into new crystal shapes and sometimes the development of foliations and lineations as described in the previous section. However, recrystallization can be due to just temperature changes without any differential pressure conditions, and when temperatures are increased, there can be a corresponding increase in mineral sizes as initially small minerals become fused into larger crystals. This fusing of numerous smaller mineral sizes into fewer, yet larger, mineral sizes is known as annealing in metallurgy, but for metamorphic rocks it is still referred to as recrystallization.
In order to understand why increasing temperatures lead to increased grain sizes, we need to address stability. In general, a mineral grain or crystal is most stable when it has a low surface area to volume ratio; therefore, large grains are more stable than small grains, since increasing the grain size results in a greater increase in volume as opposed to a smaller increase in the surface area. Stability is important because it is the reason rocks undergo metamorphism. Rocks become unstable when their environment changes, and by a recrystallization processes (metamorphism), they can return to a stable form once again. Figure 5.2 demonstrates the recrystallization process in a sedimentary rock in response to elevated temperature. In this example, the original grains are smaller and rounded, but recrystallization resulted in larger grains that are interlocking; the pore spaces are gone and instead larger crystals exist.
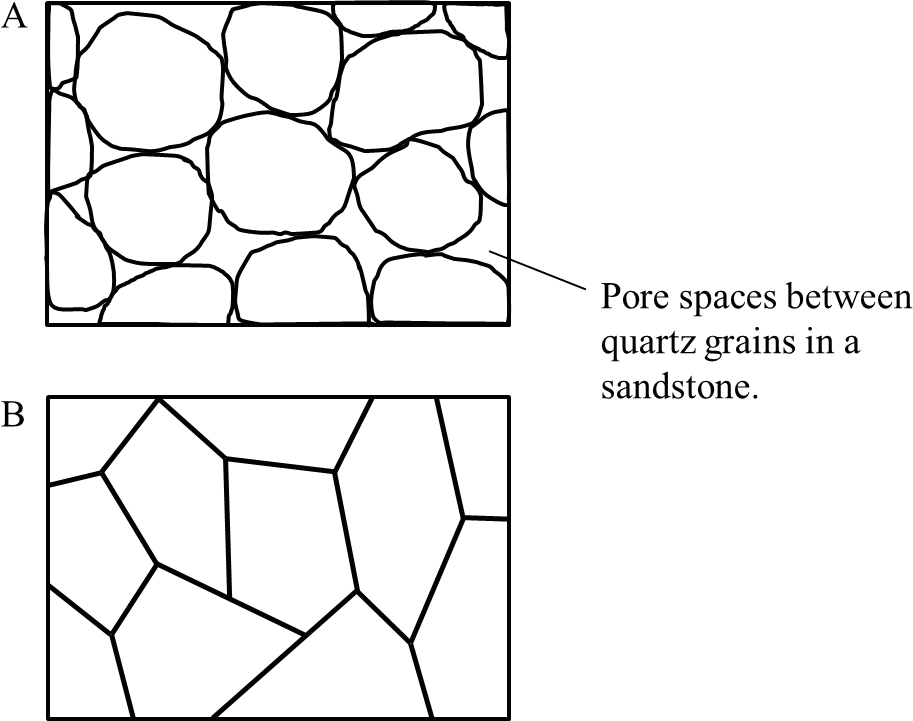
Source: Karen Tefend (2015) CC BY-SA 3.0 view source
In addition to increased grain size with increased temperature, occasionally a new mineral forms during metamorphism. These new minerals form at certain temperatures and are called index minerals, which can be used to determine the temperature of metamorphism.
5.2.3 Chemically Reactive Fluids
The phrase chemically reactive refers to the dissolved ions in a fluid phase that may react with minerals in a rock; chemical replacement occurs when these ions take the place of some atoms in the mineral’s structure, which may lead to a significant change in the rock’s chemical composition. These fluids can be quite hot, especially if they are released from a nearby igneous intrusion. Metamorphism due to such fluids is known as hydrothermal metamorphism.
5.3 METAMORPHIC ROCK NAMES
5.3.1 Foliated Metamorphic Rocks
Differential pressures can cause a metamorphic pattern known as foliation to develop in metamorphosed rocks. There are a few types of foliations that are commonly seen in metamorphic rocks, each foliation type is dependent on the minerals that define the foliation. Gneissic banding (e.g. Figure 5.3) is defined as alternating dark and light mineral bands throughout the rock; the metamorphic rock with this type of foliation is called gneiss (pronounced “nice”). In Figure 5.3A, the layering in this gneiss is horizontal, and the greatest pressures were at right angles to the gneissic bands. Note that these bands are not always flat, but may be seen contorted as in Figure 5.3B; this rock still is considered to have gneissic banding even though the bands are not horizontal. The typical minerals seen in the dark coloured bands are biotite and/or amphiboles, whereas the light-coloured bands are typically quartz or light-coloured feldspars. The protoliths for gneiss can be any rock that contains more than one mineral, such as shale with its clay minerals and clay-sized quartz and feldspar, or an igneous rock with both dark-coloured ferromagnesian minerals and light-coloured non-ferromagnesian minerals (see Chapter 3 for review). In order for gneissic foliation to develop, temperatures and pressures need to be quite high; for this reason, gneiss rocks represent a high grade of metamorphism.
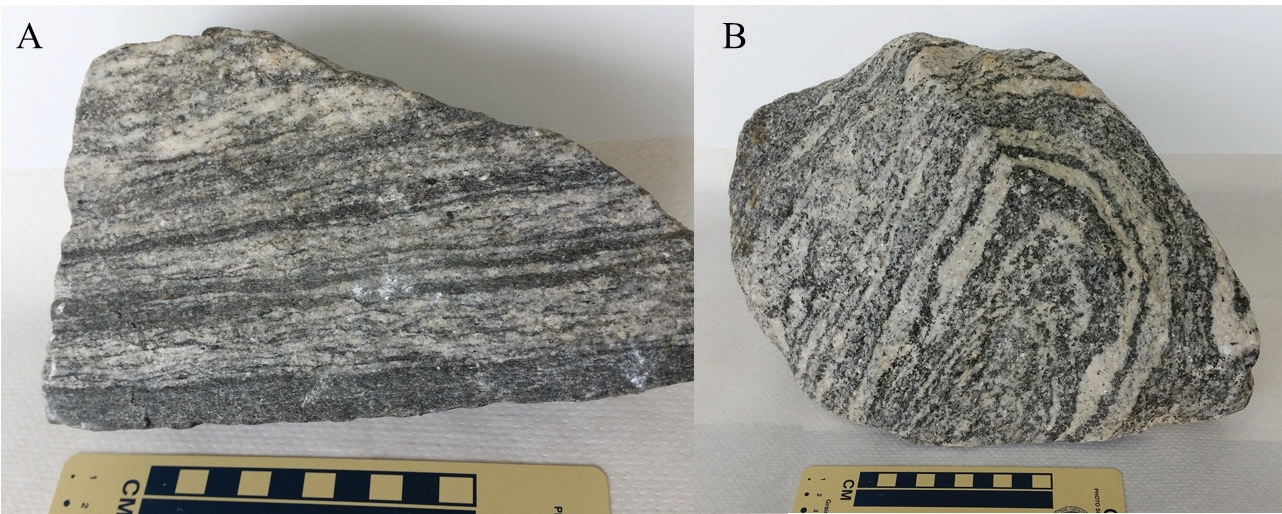
Source: Karen Tefend (2015) CC BY-SA 3.0 view source
Another type of foliation is defined by the presence of flat or platy minerals, such as muscovite or biotite micas. Metamorphic rocks with a foliation pattern defined by the layering of platy minerals are called schist; the rock name is commonly modified to indicate what mica is present. For example, Figure 5.4 is a photo of a muscovite schist, with garnet present, so the correct name for this rock is a garnet muscovite schist. By convention, when naming a metamorphic rock the mineral in the lowest quantity is mentioned first. The muscovite micas define a very wavy foliation in the rock; this textural pattern of wavy micas is called a schistose foliation (e.g. Figure 5.4B). Shale is usually the protolith for schist; during metamorphism, the very tiny clay minerals in shale recrystallize into micas that are large enough to see unaided. Temperatures and pressures necessary for schistose foliation are not as high as those for gneiss; therefore schists represent an intermediate grade of metamorphism.

Source: Karen Tefend (2015) CC BY-SA 3.0 view source
Another metamorphic rock is called phyllite, which is made up of fine crystals that give the rock a shiny appearance. This rock displays phyllitic foliation, which has good, sometimes wrinkled or folded, cleavage. Phyllites are generally formed from a shale protolith, with the clay minerals in the shale aligning and recrystallizing as small mica minerals. This foliation was formed under temperatures and pressures less than those for schist and represents a low to intermediate grade of metamorphism.
The next type of foliation is only seen in the metamorphic rock called slate, which forms by the low temperature and pressure alteration of a shale protolith. The clay sized minerals in the shale recrystallize into very tiny micas, which are larger than the clay minerals, but still too small to be visible. The alignment of these micas control how the slate breaks, and it tends to break parallel to the mica alignment. Since we are unable to see the aligned micas, we can use the alignment of the rock fracture pattern to define the foliation, as the rock is cleaved or split. This is called slaty cleavage, and a rock displaying this foliation type is called a slate. Figure 5.5 is an example of the foliated slate displaying slaty cleavage; notice that this rock has retained its original sedimentary layering, which is quite different from the foliation direction. Slate is an example of a low grade metamorphic rock, as the original sedimentary features and, occasionally, fossils may be preserved due to the low temperatures and pressures barely altering the shale protolith.
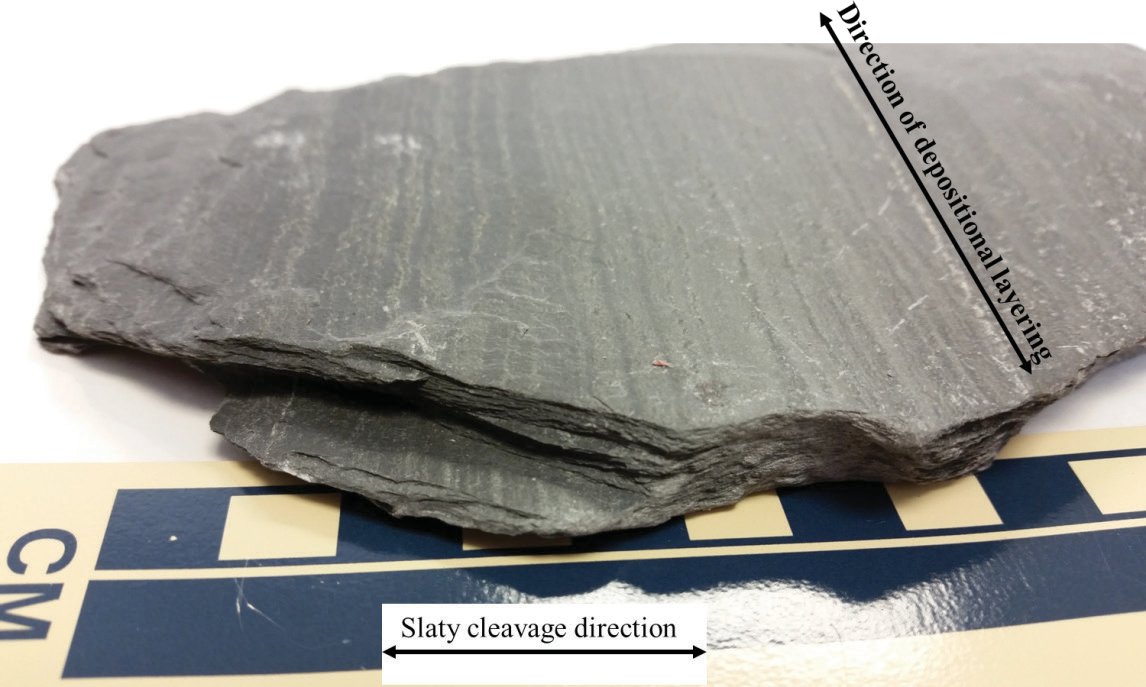
Source: Karen Tefend (2015) CC BY-SA 3.0 view source
5.3.1 Non-Foliated Metamorphic Rocks
The other class of metamorphic rocks is non-foliated; the lack of foliation may be due to a lack of differential pressure involved in the metamorphic process; however, this is not necessarily the case for all non-foliated metamorphic rocks. If the protolith rock is only composed of one mineral type, such as limestone or a sandstone with only quartz sands, then a foliation will not develop, even with differential pressure. The calcite mineral in limestone and the quartz sands in sandstone are neither platy minerals, nor are there different coloured minerals in these rocks. The calcite and quartz minerals recrystallize into equigranular, coarse crystals (e.g. Figure 5.2B), and the metamorphic rocks that they make are named by their composition, not by foliation type. For example, Figure 5.6 is quartzite, a metamorphosed quartz-rich sandstone, while Figure 5.7 shows two examples of marble; note that colour can vary for both marble and quartzite. Quartzite and marble may be hard to identify based on appearance, therefore, you must rely on the properties of the minerals that comprise these rocks; you may recall that quartz is harder than glass, while limestone is softer than glass. If you zoom in for a close view of the marble in Figure 5.7, you will see the calcite crystals are fairly large compared to the quartz crystals in the quartzite in Figure 5.6; this can be attributed to the temperature of metamorphism, as higher temperatures result in larger crystals.
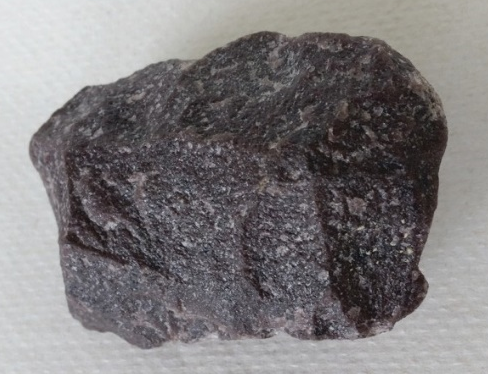
Source: Karen Tefend (2015) CC BY-SA 3.0 view source
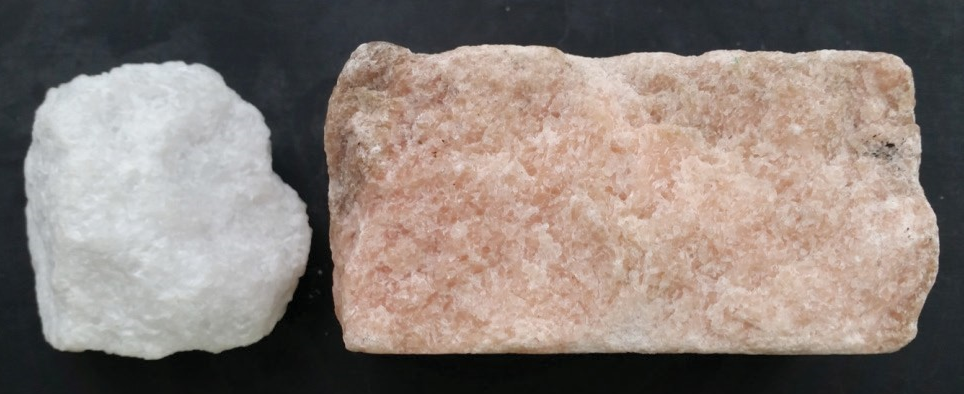
Source: Karen Tefend (2015) CC BY-SA 3.0 view source
In order to identify and name metamorphic rocks, a logical first step would be to examine the rock for evidence of any pattern or foliation, and if present, identify which minerals are making the foliation pattern. Non-foliated metamorphic rocks can be identified by the properties defined by their mineral composition. Below is a table summarizing the metamorphic rock types, foliation names, and protolith rock types.

Source: Lyndsay Hauber & Joyce M. McBeth (2018) CC BY 4.0 after Karen Tefend (2015) CC BY-SA 3.0 view source
5.4 TYPES OF METAMORPHISM
5.4.1 Regional Metamorphism
Metamorphism that affects entire rock bodies over a broad region is referred to as regional metamorphism. There is a wide range of conditions in temperature and pressure that produce a wide range of metamorphic rock types; for example, all of the foliated rocks fall into this metamorphic category and some non-foliated rocks as well. The main point is that a large area is affected by changes in temperature and/or pressure. One type of regional metamorphism occurs at convergent plate boundaries, where rocks are subjected to a variety of pressures and temperatures, resulting in a thermodynamic metamorphism (“thermo-” for increased temperature, and “dynamic” for increased pressure). Mountain ranges that form by the converging of tectonic plates are classic examples of thermodynamic metamorphism. Regional metamorphism also occurs along plate boundaries where an oceanic plate descends (subducts) back into the mantle as a result of plate convergence; oceanic plates that subduct into the mantle will form a deep ocean trench, such as the trench along the western margin of South America. With increased pressure in these regions metamorphic foliations can get quite pronounced, as we saw in the development of slate to schist to gneiss; with increased temperature we see increases in grain size as well as the introduction of new minerals in the metamorphosed rock.
5.4.2 Contact Metamorphism
As mentioned previously, recrystallization due to increased temperature results in the formation of larger minerals, or sometimes in the formation of new minerals (Figure 5.11). Contact metamorphism describes the type of metamorphism attributed to increased temperature, usually from proximity to a heat source such as an intrusive magma body or a lava flow, and the rock undergoes a thermal metamorphism. The rocks that are in closer contact to the magma will form larger crystals due to the higher heat. Rocks that are in contact with hot, chemically reactive fluids can also fall within the contact metamorphism category; recrystallization due to this type of contact is called hydrothermal metamorphism. Unlike the regional metamorphism described in the previous section, both types of contact metamorphism are confided to smaller areas near the heat source (magma, lava, or hydrothermal fluids). Since differential pressures are not involved, contact metamorphism results in the formation of non-foliated rocks. The non-foliated rock types that were covered previously included marble, quartzite, amphibolite, and anthracite coal.