4. Intracellular Signalling
Chapter 4 – Signalling Inside the Cell
- 4.1 Introduction
- 4.2 G-Protein-Coupled Receptors
- 4.2.1 G-proteins
- 4.3 The cAMP Signalling Pathway
- 4.4 The Phosphatidylinositol Pathway
- 4.5 Calcium Signalling
- 4.6 Enzyme-Linked Receptors
- 4.7 Ligand-Gated Ion Channel Receptors
- 4.8 Nuclear Transcription Factor Receptors
- 4.9 Interactions between Signalling Pathways
- 4.10 Summary
- 4.11 Glossary
4.1 Introduction
In the previous chapter, we saw how cells in the body send chemical signals to each other. The distances involved can be a small as the 20-nanometre gap of a synapse, or as far as the nearly 2 metres between the pituitary gland and the toe – In humans, that is! In the blue whale, the tail can be more than 20 metres from the pituitary gland.
How do these extracellular signals get into the cell?
The answer is simple for extracellular signalling molecules that do not form multiple strong electrostatic bonds with water molecules, because they can easily leave the aqueous environment to diffuse freely through the lipid bilayer of the cell membrane and enter the cytoplasm. Examples of signalling molecules that rapidly cross the plasma membrane are steroid hormones and nitric oxide (NO).
The answer is more complicated for signalling molecules that are hydrophilic and form multiple electrostatic bonds with water. Hydrophilic molecules are molecules that water can grab onto. They cannot cross the lipid bilayer because that would require breaking multiple bonds with water at the same time. Therefore, the cell surface has to recognize and bind these signalling molecules, and use other molecules to pass the signal to the inside of the cell.
In both cases, the extracellular signalling molecule binds to a specific receptor protein on or in the target cell. The signal molecule (ligand) causes a change in the shape or arrangement of the receptor protein. This change in receptor shape or configuration passes the signal along. Receptors are located either on the cell membrane or inside the cell – usually an intracellular receptor in the case of lipophilic signalling molecules, and a transmembrane receptor in the case of hydrophilic signalling molecules. Membrane receptors include the G protein-coupled receptors (GPCRs), the enzyme-linked receptors, and the ligand-gated ion channel receptors. Intracellular receptors include the steroid and thyroid hormone receptors. The steroid and thyroid hormone receptor proteins act as transcription factors in the nucleus.
Amplification is an important concept in this chapter. The chemical signals in the body typically involve only very low concentrations of the signalling molecules. To translate changes of only a few signalling molecules into big changes in the activity of cells and organs, cells often use more than one chemical reaction in a sequence of steps. Researchers often call such a chain of signalling events a “signal transduction cascade”.
Each step in a signal transduction cascade can greatly amplify a signal, because a small number of molecules can affect the production of many downstream molecules through enzymatic activity. Amplification also means that signal transduction doesn’t need to use much energy, since a few molecules are all that are needed to carry a signal.
We’ve already touched on these topics in the previous chapter, so now it’s time to look at a few intracellular signalling pathways in greater detail.
4.2 Signalling Through the GPCRs
The G protein-coupled receptors are a large family of cell membrane receptors: Animals typically have hundreds of different GPCRs, each binding and recognising a different ligand. Ligands that bind and activate GPCRs vary in size, from small amines to large, complex proteins. These ligands include hormones, neurotransmitters, light-sensitive compounds, odour molecules, and pheromones.
GPCRs derive their name from their association with a family of signalling proteins called the G proteins. G-proteins are called G proteins because they bind the guanine ribonucleotides guanosine diphosphate (GDP) and guanosine triphosphate (GTP). G-proteins function as molecular switches, turning the activity of downstream effectors on and off. The G proteins that bind to GPCRs (not all do) are anchored in the inner leaflet of the cell membrane.
GPCRs have an extracellular amino-terminal domain, a transmembrane domain composed of seven α-helices through the cell membrane, and an intracellular carboxyl-terminal domain. Because of the seven transmembrane α-helices, GPCRs are sometimes also called seven transmembrane receptors. The recognition site for the ligand is exposed to the extracellular face, the group of seven hydrophobic transmembrane helices anchors the protein into the membrane phospholipid, while the intracellular face has the recognition site for the G-proteins.
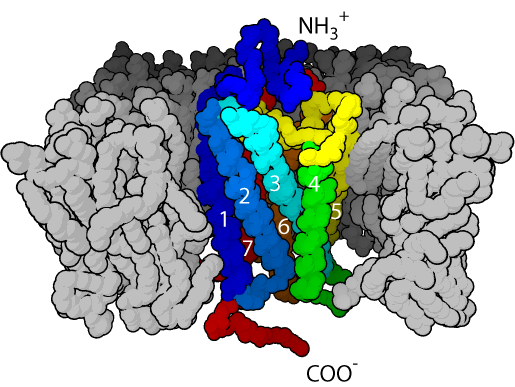
4.2.1 G-Proteins
G-proteins are an extensive family of enzymes characterized by the ability to promote hydrolysis of GTP. You can think of a G protein as a simple on-off switch: G protein carrying a GTP molecule is in its “on” state, and G protein carrying a GDP molecule is in its “off” state. The G-proteins that associate with GPCRs form complexes of three different protein subunits: α, β, and γ – named after the first three letters of the Greek alphabet. A complex with three different subunits is called a heterotrimer (Greek, hetero: “other, different”; tri-: “three”; méros “part”), so these G proteins are called heterotrimeric G-proteins. This complex couples the GPCR to enzymes located on the internal side of the plasma membrane that function as signal effectors. Heterotrimeric G proteins usually stick to the inner surface of the plasma membrane, which makes it easier for them to find their GPCR partners and other membrane proteins they need to interact with. Gα and Gγ are typically modified with hydrophobic lipid sidechains that can insert themselves into the inner leaflet of the plasma membrane’s lipid bilayer, and all three subunits, α, β, and γ, have positively-charged amino acid sidechains that bind to the negatively-charged headgroups of membrane phospholipids.
In the basal state, G-proteins are bound to GDP. Upon ligand binding to the receptor, the G-protein α-subunit loosens its grip on GDP; GDP dissociates from the α-subunit, allowing GTP to take its place. In that way, the GPCR converts inactive Gα-GDP into activated Gα-GTP. Both Gα-GTP and the dissociated Gβγ complex can either stimulate or inhibit a variety of effectors, including enzymes that generate second messengers and ion channels.
The α-subunit possesses an intrinsic enzymatic activity (GTPase); it hydrolyzes GTP into GDP and inorganic phosphate (Pi) in a few seconds, making the α-GTP unstable and causing the complex to re-associate into the inactive basal state form, αβγ. Under this condition the αβγ complex deactivates the receptor. In this way, the GPCRs serve as allosteric regulators of G-protein activity, switching between active and inactive states of the G-protein.
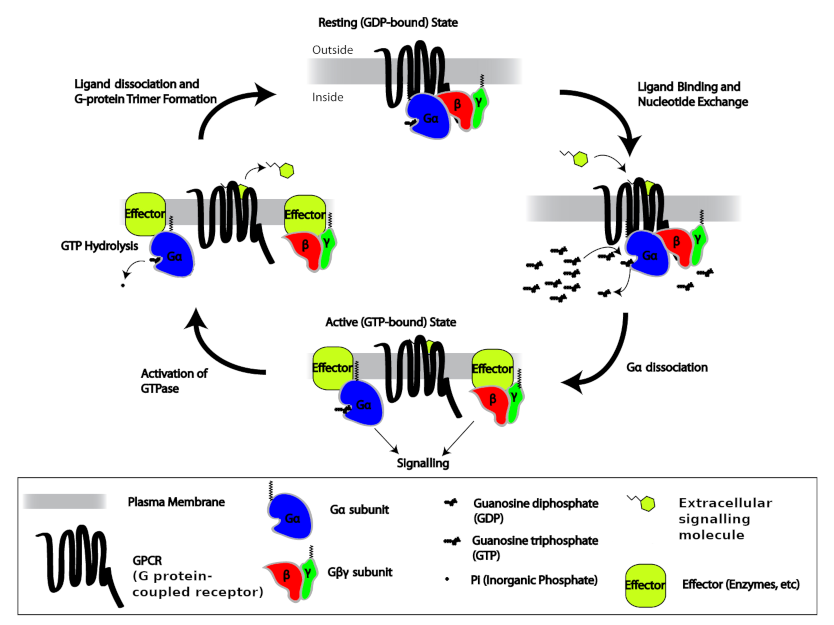
Several sub-classes of heterotrimeric G-proteins have been described. However, for the purpose of this text only those associated with three different signal effectors, mediating three different responses, will be described:
- Gαs – or G-stimulatory, associated with the effector enzyme adenylyl cyclase (AC), a transmembrane glycoprotein that catalyzes the conversion of ATP into the second messenger cAMP;
- Gαi/o – or G-inhibitory, also associated with AC but with inhibitory, instead of stimulatory, effects on the synthesis of cAMP; and
- Gαq/11 – associated with the effector enzyme phospholipase C-β (PLCβ), a transmembrane protein that hydrolyses the membrane phospholipid phosphatidylinositol 4,5-biphosphate (PIP2) to make the second messengers inositol (1,4,5) triphosphate (IP3) and diacylglycerol (DAG).
Based on the association of the G-protein with their effectors, two main signalling pathways involving the GPCRs can be distinguished, the stimulatory and the inhibitory cAMP signalling pathways, and the phosphatidylinositol signalling pathway.
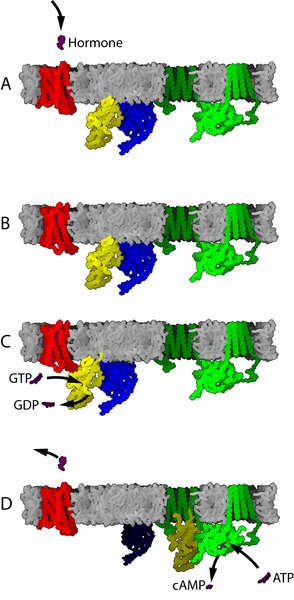
4.3 The cAMP Signalling Pathways
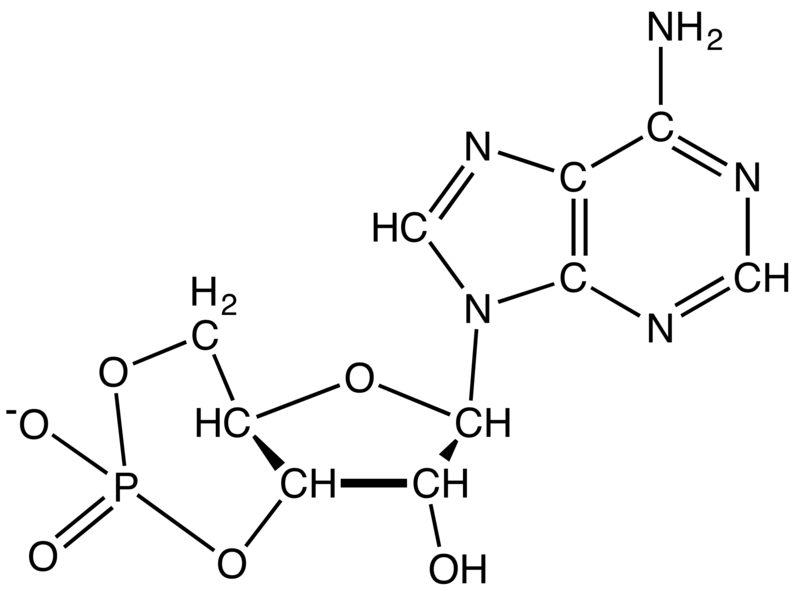
The cAMP signalling pathways – also referred to as cAMP-dependent pathways or protein kinase A pathways – can be either stimulatory or inhibitory of a particular cell function, depending if the receptor is associated with the stimulatory G-protein, Gαs (GPCRs) or to the inhibitory G-protein Gαi (GPCRi). The cAMP signalling pathway contains other components including:
- The effector enzyme adenylyl cyclase that generates the second messenger cAMP
- The enzyme protein kinase A (PKA); and
- The enzyme cAMP phosphodiesterase.
Adenylyl Cyclase, and cAMP
Adenylyl cyclase (AC) – also known as adenylate cyclase – is a transmembrane protein in the cell membrane that catalyzes the conversion of ATP into cyclic adenosine monophosphate (3′-5′-cyclic adenosine monophosphate, cAMP or cyclic AMP). cAMP is an adenine nucleotide in which one phosphate group is esterified to both the 3′- and 5′-positions of the ribose sugar part of the nucleoside.
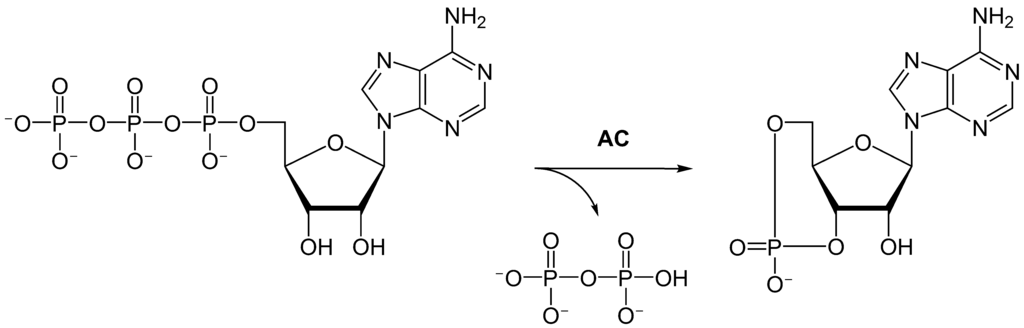
AC is the effector of both the Gαs and Gαi; interaction of Gαs with AC stimulates generation of cAMP, while interaction of Gαi with AC inhibits generation of cAMP. Thus, a GPCR coupled to Gαs counteracts the actions of a GPCR coupled to Gαi, and vice versa.
4.3.2 Protein Kinase A (PKA)
PKA is a cAMP-dependent serine/threonine specific kinase. The PKA holoenzyme is composed of two regulatory (R) and two catalytic (C) subunits. Under low levels of cAMP, PKA remains as an inactive enzyme. Upon signalling stimulation of GPCR, the concentration of cAMP rises and cAMP binds to the two binding sites on the regulatory subunits of PKA leading to the release of its catalytic subunits. The free catalytic subunits of PKA can then catalyze the transfer of ATP terminal phosphates to protein substrates at serine, or threonine residues of other kinases, resulting in a change in activity of selected intracellular proteins of the PKA pathway.
PKAs are present in a variety of cells and act on different substrates involved in many different pathways. PKA phosphorylates a variety of cytoplasmic proteins initiating a cascade of kinase reactions that amplify the original extracellular signal’s magnitude by several orders. Through phosphorylation, PKA can directly increase or decrease the activity of a variety of cytoplasmic proteins. PKA can also phosphorylate nuclear proteins, including the transcription factor CREB involved in transcriptional regulation of several genes.
4.3.3 cAMP Phosphodiesterases
cAMP is inactivated by enzymes called nucleotide phosphodiesterases (PDEs), that hydrolyze cAMP into the inactive metabolite 5′-adenosine monophosphate (5’AMP). Thus, PDEs regulate the levels of cAMP, and therefore the overall activity of the PKA pathway. The PDEs belong to a large family of mammalian proteins, constituted of at least 20 different members that contain a central catalytic domain and an N-terminal regulatory domain that binds the complex Ca2+-CaM and the second messenger cGMP (described below).
4.4 The Phosphatidylinositol (PIP) Signalling Pathway
The effector of Gαq is phospholipase C-β (PLCβ), an enzyme that catalyzes the cleavage of membrane-bound phosphatidylinositol 4,5-biphosphate (PIP2) into the second messenger’s inositol (1,4,5) triphosphate (IP3) and diacylglycerol (DAG). IP3 binds to specific calcium channel receptors located in the membrane of the endoplasmic reticulum (ER) to elicit Ca2+ release from the ER, while DAG diffuses along the plasma membrane where it may activate membrane localized forms of a second serine/threonine kinase called protein kinase C (PKC). PKC phosphorylates many other proteins, changing their activities, leading to a wide range of cellular responses. Ca2+ also plays an important role in the PIP pathway because it cooperates with DG in activating PKC.
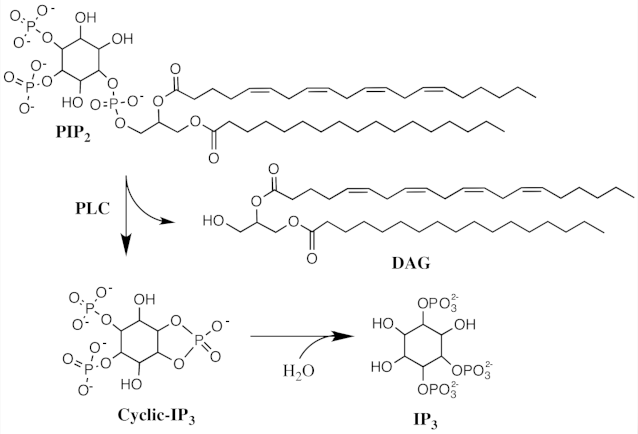
4.5 Calcium Signalling
The calcium ion (Ca2+) is also a second messenger of intracellular signalling pathways. It is liberated from the endoplasmic reticulum (ER) almost instantly upon binding of an extracellular signalling molecule to GPCRs. ER calcium pumps return the calcium to the interior (lumen) of the ER, limiting the duration of the calcium signal in the cytoplasm and maintaining a basal level of calcium in the cytosol approximately one thousand fold lower than in the extracellular fluid. Once in the cytoplasm, Ca2+ binds to the small messenger protein calmodulin (abbreviation for CALcium-MODULated proteIN; CaM). CaM transduces calcium signals by changing its folded specific three-dimensional shape upon binding to Ca2+ (Ca2+-CaM). The change in calmodulin’s conformation modifies its interactions with multiple target proteins, allowing calmodulin to act as a signalling intermediate in many different functions in the cell.
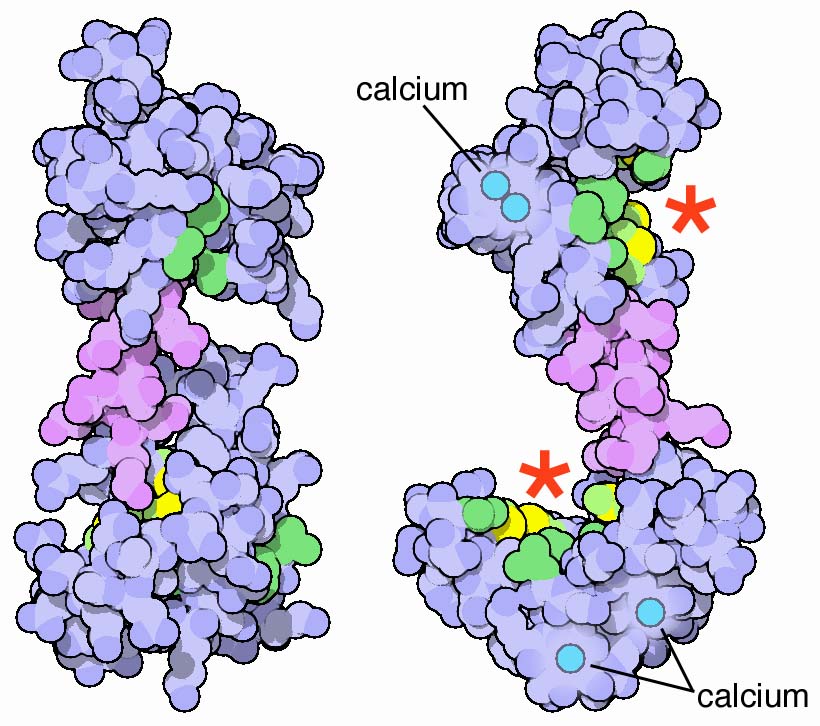
One of the most important proteins that Ca2+-CaM binds to is Ca2+/calmodulin-dependent protein kinase II (CaM kinase-II), a serine/threonine-specific protein kinase involved in many signalling cascades. Binding of Ca2+-CaM to CaM kinase-II activates CaM kinase-II, causing it to phosphorylate many different proteins of the cell. CaM kinase-II also phosphorylates itself (autophosphorylates) upon Ca2+-CaM binding, which allows CaM kinase-II to continue to remain active for a defined time period after the initial Ca2+ signal is gone. CaM kinase-II is one of the most abundant proteins in brain tissue, and is important in memory and learning, but CaM kinase-II and similar Ca2+-CaM-dependent protein kinases play important signalling roles in every tissue of the body.
Ca2+ regulates adenylyl cyclase and phosphodiesterase in the cAMP signal pathway, and cooperates with DAG in activating PKC. Thus, the cAMP signalling pathway and the PIP signalling pathways are connected together by Ca2+-CaM. This is an example of cross-talk between signalling pathways (see Section 4.9 in this chapter).
4.6 Signalling Through Enzyme-Linked Receptors
Enzyme-linked receptors are single-span transmembrane proteins with two functions carried by the same molecule: It binds the ligand; and serves as the cell membrane signal effector.
The effector is a kinase that transfers phosphate groups to specific amino acid residues of the receptor. Enzyme-linked receptors mediate the effects of some extracellular messengers that fit into the definition of classical hormones, however, most of their ligands are local regulators that act in a paracrine, autocrine, or juxtacrine mode of signalling (Chapter 3). Although enzyme-linked receptors were originally classified as typically slow response mediators of extracellular signals, it is now known that they can also mediate direct and rapid changes on gene expression that affects the cytoskeleton, and regulate cell growth, shape, and movements. Enzyme-linked receptors can be classified into four classes:
- Tyrosine kinase;
- Tyrosine kinase-associated;
- Serine/threonine kinase and
- Guanylate cyclase-associated.
4.6.1 Tyrosine Kinase Receptors
Tyrosine kinase receptors are single transmembrane ligand binding proteins with the capability of phosphorylating their own tyrosine residues (autophosphorylation) of the cytosolic region of the molecule. Tyrosine kinase receptors are activated by many of the hormones and growth factors involved in cell growth, proliferation, differentiation, and survival. Receptor activation involves interaction of two adjacent receptor molecules, forming a dimer that cross-links in the cell membrane. Dimerization brings the kinase domains of both molecules into close proximity, allowing autophosphorylation. The mechanism of dimerization varies depending on the ligand-receptor system.
4.6.2 Tyrosine Kinase-Associated Receptors
Tyrosine kinase-associated receptors are transmembrane proteins which lack intrinsic enzymatic activity, but are associated with cytoplasmic tyrosine kinases by non-covalent bonds. Tyrosine kinase-associated receptors are composed of two or more ligand specific polypeptide chains that form dimers. Upon ligand binding the dimers phosphorylate their respective tyrosine residues, as well as the residues of other signalling proteins that activate a group of cytoplasmic enzymes termed Janus kinases (JAKs; named after the two-faced Roman god because the protein has two copies of the catalytic domain). Some hormones, such as growth hormone (GH), prolactin (PRL), and erythropoietin, mediate their effects through tyrosine-kinase-associated receptors.
4.6.3 Serine/Threonine Kinase Receptors
Serine/threonine kinases receptors are single-chain peptides with a large extracellular domain containing a cysteine-rich region, a transmembrane domain, and a cytoplasmic domain. The cytoplasmic domain contains two separated amino acid sequences homologous to other serine threonine kinases. Several members of the serine/threonine kinase receptors have been characterized, including the receptors for transforming growth factor (TGF) β-I and β-II, activin, inhibin, Müllerian-inhibiting substance (MIS), and bone morphogen (BMP).
4.6.4 Guanylate Cyclase-Associated Receptors
Guanylate cyclase associated receptors mediate effects by activating the enzyme guanylate cyclase (GC), through a mechanism that has some analogies with adenylate cyclase, since it catalyzes the conversion of GTP into the second messenger cyclic cGMP. Several extracellular regulatory molecules, including atrial natriuretic peptide (ANP), also known as atrial natriuretic factor (ANF), and the gases nitric oxide (NO) and carbon monoxide (CO) mediate their effects by activating GC. Like the tyrosine and serine/threonine kinases, GC can directly be both receptor and effector.
Two main types of GC have been described, a membrane associated form (mGC) and a cytoplasmic soluble form (sGC) that directly catalyze the production of cGMP in the cytosol. ANP, guanylin, and the sea urchin sperm proteins interact with mGC, whereas NO and CO interact with sGC. NO is synthesized from L-arginine by the action of NO-synthase and is induced in various tissues by cytokines and endotoxins. One of the most prominent actions of NO is vascular smooth muscle relaxation in the penis. cGMP can activate cGMP-dependent protein kinases (cGMP-PK) and stimulate the actions of specific phosphodiesterases, which in turn inactivate cGMP and cAMP.
cGMP has a number of functions, including relaxation of smooth muscle, inhibition of platelet activation, reduction of endothelial permeability, and weakening of heart muscle contractions (inotropic effect, not to be confused with ionotropic effect).
4.7 Signalling Through Ligand-gated Ion Channels
Ligand-gated ion channel receptors (LGIC), also termed ionotropic receptors, are ion-selective transmembrane channels formed by multi-subunit proteins. LGIC are activated and opened upon binding of several neurotransmitters, among others acetylcholine (ACh), γ-aminobutyric acid (GABA), glycine, glutamate and serotonin (5-hydroxytryptamine; 5-HT3).
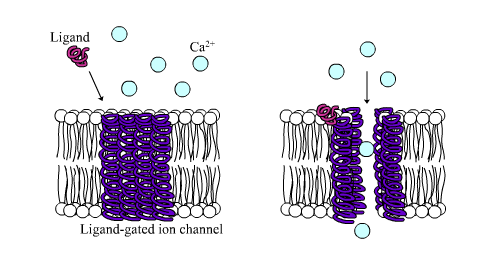
The most thoroughly studied LGIC receptor is the nACh-R, which was first characterized in the Torpedo californica, an electric ray native to the eastern Pacific Ocean. The nACh-R is composed of 12 different glycosylated subunits, α2 through α10 and β2 through β4. These subunits combine to form pentamers that are similar to one another, mainly in the hydrophobic domains. The muscle nACh-R is composed of two α-subunits, one β-subunit, one δ-subunit, and either one γ-subunit or one ε-subunit. The neuronal forms of the nACh-R are more heterogeneous with a larger number of subunit combinations. The subunits are organized into a pentagonal array forming a pore that crosses the membrane.
The structure of the ligand-gated ion channel receptors can be described as follows:
- Each subunit is composed of four hydrophobic α-helical domains and each domain spans the plasma membrane four times;
- The α-subunits contain the ligand-binding site, thus, activation of the receptor requires binding of two molecules of the ligand;
- A large extracellular amino-terminal domain with multiple sites for glycosylation, together with one of the α-transmembrane domains in each subunit (M2), lines the pore for the passage of ions;
- A short extracellular carboxy-terminal domain; and
- A large intracytoplasmic loop, located between transmembrane regions 3 and 4, contains multiple potential phosphorylation target sites, suggesting that protein kinases may regulate this receptor activity.
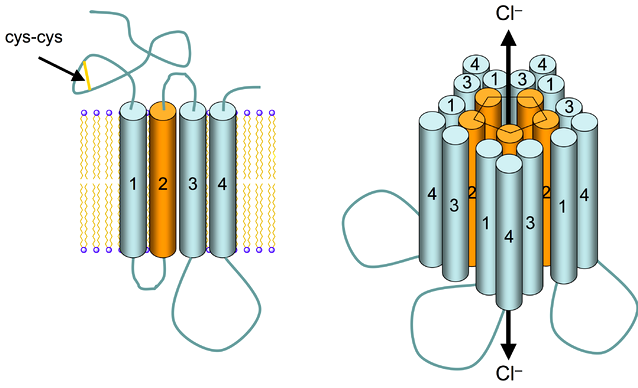
The channel part of the receptor is constituted by a structure of several rings of specific amino acids. An outer hydrophilic ring is followed by two hydrophobic rings, one of valine and the second of leucine, and by two serine threonine rings. The serine-threonine rings are the narrowest part of the channel and function as a gate. Upon ACh binding, the M2 rotates in a way that leucine side chains move to the side, enlarging the pore size like a diaphragm. The structures of the other known ligand-gated ion channel are similar to that of the nACh-R, suggesting that they may have derived from a common ancestral gene.
The main functional feature of this group of receptors is that they do not depend on intracellular mediators; therefore, they can convey a signal rapidly into the cell. However, the LGIC are also regulated by the G-proteins. The mACh-R-regulated K+ channel and the β-adrenergic-gated L-type Ca2+-channel found in the heart are regulated by the βγ and αs G-proteins, respectively.
ACh has two types of receptors: the muscarinic ACh receptors (mACh-R), which are GPCRs, and the nicotinic ACh receptors (nACh-R), also called nicotinic cholinergic receptors. In muscle cells the nicotinic ACh receptor is a ligand-gated Na+ channel, while in neurons the the nicotinic ACh receptor is a ligand-gated Ca2+-channel.
5-hydroxytryptamine (5-HT) and GABA mediate their effects through GPCRs and the LGIC. However, 5-HT3 also binds to a divalent cation channel receptor, while GABA binds to the GABAA and GABAC receptors that are Cl- channels.
4.8 Signalling Through Nuclear Transcription Factor Receptors
Nuclear transcription factor receptors comprise a large family of intracellular single subunit phosphoproteins that interact with membrane-soluble signalling molecules and directly affect gene transcription. They are also called ligand-activated zinc (Zn)-finger nuclear receptor transcription factors because they contain one atom of Zn that interacts via coordination bonds with four cysteine residues. These receptors can recognize specific DNA consensus sequences in the regulatory regions of their target genes. Members of this family include the receptors for steroid hormones, Vitamin D, thyroid hormones, and retinoic acid.
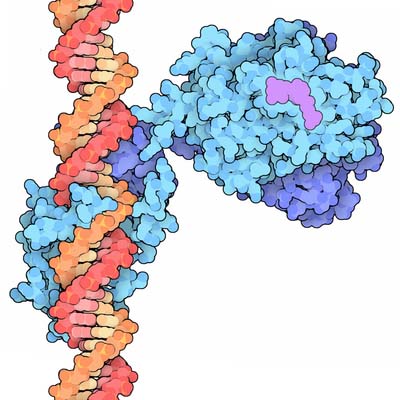
Nuclear receptor transcription factors bind to specific nucleotide sequences found in the regulatory region of target genes, termed hormone response elements (HRE). Nuclear receptors transcription factors binds as dimers, in which each monomer recognizes only half of the consensus sequence. Thus, the orientation and spacing between half-sites of the HRE determines receptor specificity. Three different regions can be distinguished in the nuclear receptor transcription factors:
- The DNA-binding domain – characterized by a nonconserved amino-terminal region of variable size that determines tissue specificity;
- The activation domain – characterized by a conserved central DNA-binding region that contains basic amino acids and 9 conserved cysteine residues. Two Zn-finger substructures can be distinguished in this domain, each containing one molecule of Zn that interacts via coordination bonds with four cysteine residues. The two Zn fingers are flanked by α-helices at the carboxy-terminal regions, which include a proximal box (P-box) and a distal box (D-box). Both Zn-fingers are structurally and functionally different and are encoded by two different exons. The α-helix of the amino-terminal-proximal Zn finger interacts directly with the major groove of the DNA. Three amino acids – glycine, serine, and valine – at the amino-terminus of the α-helix, are responsible for the recognition of a DNA responsive element. The second Zn-finger appears to be involved in protein-protein interactions such as receptor dimerization and stabilization of complexes; and
- The hormone-binding domain – characterized by a carboxy-terminal region, of approximately 250 amino acids that recognize and bind the ligand with high affinity.
Steroid hormone receptors are complexed to the chaperone heat shock protein (HSP). Upon ligand binding, the steroid receptor undergoes phosphorylation of its serine residues at the amino-terminal activation domain, and dissociates from the HSP to become activated. The activated receptors form dimers and bind to palindromic steroid-responsive cis-acting elements located upstream of the CAAT and TATA boxes of the genes. DNA-dependent RNA-polymerase-II and other transcription factors and coactivators are then recruited to initiate transcription of the steroid response genes.
4.9 Interactions Between Signalling Pathways
Any given cell is under the influence of more than one extracellular regulatory molecule at one time. Several extracellular regulatory molecules, including hormones, neurotransmitters, and growth factors, activate more than one pathway at the same time. Therefore, any particular signalling cascade typically operates under conditions where other signalling pathways are simultaneously active in the same cell. There are a few different reasons for this. For instance, the endocrine and nervous systems have more than one way to achieve any given biological response. Organs and cells have an elaborate regulatory capacity with overlapping controls. Redundant systems may have evolved as fail-safe mechanisms to secure vital functions: If one signalling pathway fails, another signalling pathway can act as a back-up to ensure survival. Redundant systems probably also evolved to fine-tune responses: In physiology, one often encounters signalling systems that have similar, but not identical, functions.
Signalling pathways can respond to the same agonist, or to different agonists, interacting to affect similar intracellular processes. These interactions are called “cross-talk”, in which two or more distinct pathways affect the same response and produce an additive or synergistic response, or that the activation of one pathway may inhibit the response stimulated by the other.
4.10 Summary
Intracellular signalling pathways organize extracellular signals, and amplify those signals such that a small number of ligands affect the activity of a large number of molecules inside the cell. The cell’s responses to the signal can be rapid and short-lived, or they can be gradual and long-lived through the regulation of gene expression. Signalling via GPCRs involves the activation of heterotrimeric G-proteins that couple receptors with effectors. The protein kinase A (PKA) and the phosphatidylinositol (PI) phosphate pathways are examples of major intracellular pathways. The effector of the PKA pathway is adenylate cyclase which converts ATP into cAMP. The PI pathway is activated mainly by extracellular signals that activate phospholipase C (PLC), leading to the conversion of PI(4,5)-P2 into IP3 and diacylglycerol (DAG).
IP3 binds to receptors on the endoplasmic reticulum (ER) and opens ER Ca2+ – channels, inducing a rapid release of the stored Ca2+ into the cytoplasm, while DAG stimulates PKC. Enzyme-linked receptors are single span transmembrane proteins. Binding of the ligand to the extracellular domain of the receptor leads to the activation of an enzyme on the intracellular side of the membrane. The enzyme that acts as a signal effector can be a domain of the same receptor protein, or it can be a second protein that binds to the receptor. Enzyme-linked receptors are classified into four classes: tyrosine kinase, tyrosine kinase-associated, serine/threonine kinases, and guanylate cyclase-associated. Most of the ligands of the enzyme-linked receptors are local regulators and growth factors. Nuclear transcription factor receptors are intracellular proteins that bind membrane-soluble signalling molecules and directly affect gene transcription. They are classified as zinc-finger proteins because they contain a zinc ion that stabilizes the protein’s three-dimensional folding structure due to coordination bonds between the zinc atom and four cysteine residues. These receptors recognize specific DNA consensus sequences in the regulatory regions of their target genes, and binding of the ligand-activated receptor activates transcription of most target genes, though in some cases the receptor represses transcription of a target gene. Members of the nuclear receptor family include the receptors for steroid hormones, Vitamin D, thyroid hormones, and retinoic acid.
4.11 Glossary
AC – adenylate cyclase, also known as adenylyl cyclase or adenyl cyclase
adenylate cyclase –
adenylyl cyclase –
ACh – acetylcholine
ACTH – adrenocorticotropic hormone
allosteric activator – is a regulator of an enzyme that bind to an effector molecule at a site other than its active site
AMP – adenosine monophosphate
amplification –
ANP – atrial natriuretic peptide
ATP – adenosine triphosphate
BMP – bone morphogenetic protein
Ca2+ – calcium ions
Ca2+-CaM – Ca2+ bound to CaM
calmodulin –
CaM – calmodulin
cAMP – cyclic adenosine monophosphate
cGMP – cyclic guanosine monophosphate
cGMP-PK – cGMP-dependent protein kinase
CO – carbon monoxide
CREB – cAMP response element binding protein
cyclic adenosine monophosphate –
DAG – 1,2-diacylglycerol
diacylglycerol –
EGF – epidermal growth factor
EGF-R – EGF receptor
enzyme-linked receptor –
ER – endoplasmic reticulum
ERK – extracellular signal regulated kinase
FGF – fibroblast growth factor
FSH – follicle-stimulating hormone
G-protein –
G protein-coupled receptor –
GABA – γ-aminobutiric acid
Gαi –
Gαq –
Gαs –
GC – guanylate cyclase
GDP – guanosine diphosphate
GH – growth hormone
GnRH – gonadotropin releasing hormone
GTP – guanosine triphosphate
Gαi – G-protein inhibitory α-subunit
Gαs – G-protein stimulatory α-subunit
5-HT – 5-hydroxytryptamine, more commonly known as serotonin
IGF-I – insulin-like growth factor-I
IGF-II – insulin-like growth factor-II
inositol triphosphate –
IP3 – inositol triphosphate
kinase – an enzyme that transfers phosphate groups from high-energy donor molecules, such as ATP, to specific target substrates
L-type – long lasting VSCC
LGIC – ligand-gated ion channel
ligand-gated ion channel receptor –
mACh – muscarinic acetylcholine receptor
MAPK – mitogen-activated protein kinase
MEK – MAP-kinase-kinase or MAPKK
MIS – Müllerian-inhibiting substance
MSH – melanocyte-stimulating hormone
nACh-R – nicotinic acetylcholine receptor
NO – nitric oxide
nuclear receptor –
nucleotide phosphodiesterase –
PDE – nucleotide phosphodiesterase
PDGF – platelet-derived growth factor
phosphatidylinositol –
phosphatidylinositol 4,5-biphosphate –
phosphodiesterase – an enzyme that cuts phosphodiester bonds. Phosphodiesterase enzymes are important in intracellular signalling because they inactivate cyclic nucleotides such as cAMP and cGMP.
phospholipase C –
phosphorylation –
PI – phosphatidylinositol
PI-PLC – phosphatidylinositol-specific phospholipase-C
PI(3,4,5)P3 – phosphatidylinositol 3,4,5-triphosphate
PI(3,4)P2 – phosphatidylinositol 3,4-biphosphate
PI(4,5)P2 – phosphatidylinositol 4,5-biphosphate
PKA – cAMP-dependent protein kinase-A
PKC – protein kinase-C
PLC – phospholipase-C
PRL – prolactin
protein kinase A –
seven transmembrane receptor –
signal transduction cascade –
TK – tyrosine kinase
transcription factor –
TSH – thyrotropin, or thyroid-stimulating hormone
TSH-R – TSH receptor
VD-R – vitamin D receptor
VGCR – voltage-gated channel receptors
VSCC – voltage-sensitive Ca2+ channel