3. Intercellular Communication
Chapter 3 – Principles of Intercellular Communication
- 3.1 Introduction
- 3.2 Long-Distance Signalling in the Animal Body
- 3.3 Neural Signalling
- 3.4 Neurotransmitters
- 3.5 Humoral Signalling
- 3.6 Extracellular Signalling Molecules
- 3.7 Intracellular Signalling
- 3.8 Cell Surface Receptors
- 3.9 Nuclear Transcription Factor Receptors
- 3.10 Signalling Through the Senses
- 3.11 Summary
- 3.12 Glossary
- 3.13 Suggested Readings
3.1 Introduction
Cells constantly communicate with each other. They communicate not only with their immediate neighbours in a tissue, but also with cells in distant organs elsewhere in the body. They even communicate with cells of other individuals. Whether we’re talking about the very simplest bacteria, or about cells in the human body, almost all cells communicate with each other using chemical signals.
Within an animal’s body, changes in the concentrations of signalling molecules are by far the most common form of communication. A second mode of communication within an animal’s body is signalling through electrical charges across the cell membrane. With the exception of some kinds of sponges, nearly all animals use electrical communication, in addition to the chemical communication found in all animals. In this chapter, we introduce some of the “how” (the mechanisms) and “why” (the functions) of communication between cells.
3.2 Long-Distance Signalling in the Animal Body
Most of the long-distance signalling that happens inside an animal’s body falls into two categories:
- Neural Signalling – For responses that need to be a lot faster than one minute, the body relies on electrical signalling. Electrical signals have one advantage over purely chemical signals: Changes in the electromagnetic field can travel much faster than individual molecules. Specialized, elongated cells called neurons (Chapter 2) carry electrical signals in the form of action potentials, and secrete chemical signals called neurotransmitters into the tiny extracellular space in the contact site between the neuron and its target cell. This contact site is called the synapse (Greek: sun-, “together”; háptō, “join”). The information sent by the nervous system travels very fast, and the responses are typically short-lived.
- Humoral Signalling – The term “humoral” (Latin, humor: liquid) refers to the extracellular body fluids: blood, lymph, cerebrospinal fluid, and the interstitial fluid between cells. Hormones are chemical signals that are liberated into the extracellular fluid to circulate through the blood stream. This chemical signalling through the circulatory system is called the endocrine system. The speed of endocrine signalling is limited by the rate of blood flow: In humans, it takes roughly one minute for the blood to complete one circuit around the body. The responses are prolonged, lasting from a few hours up to weeks. Other humoral chemical signals act over a much shorter range, as described later in Section 3.5, and can result in more rapid responses.
These two categories overlap: In some neurons, the axon doesn’t form synapses with other cells. Instead, the axon releases a chemical signal directly into the bloodstream. This is called neuroendocrine, or neurohumoral, signalling.
In addition to long-range communication, cells communicate with other over shorter distances as well. Cells in every tissue send chemical signals directly to their neighbours without necessarily forming a synapse. And in many tissues, cells can also communicate electrical signals and intracellular chemical signals via gap junctions. Chemical signals embedded in the extracellular matrix (ECM) also play an important role in communication in the animal body.
3.3 Neural Signalling
As mentioned in Chapters 1 and 2, neurons are cells that are specialized for electrical and chemical signalling to and from the cells that they come into close contact with. The physical contacts[1] that neurons make with their target cells are called synapses. The target cell can be another neuron, or it can be another cell type such as a muscle cell or a gland cell.
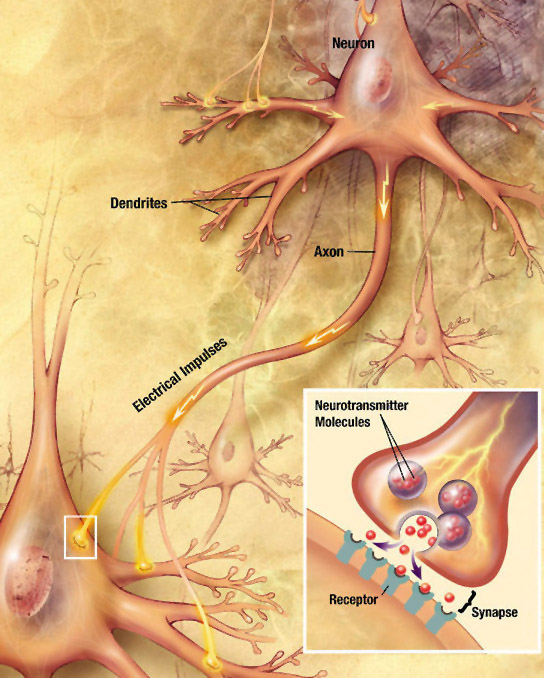
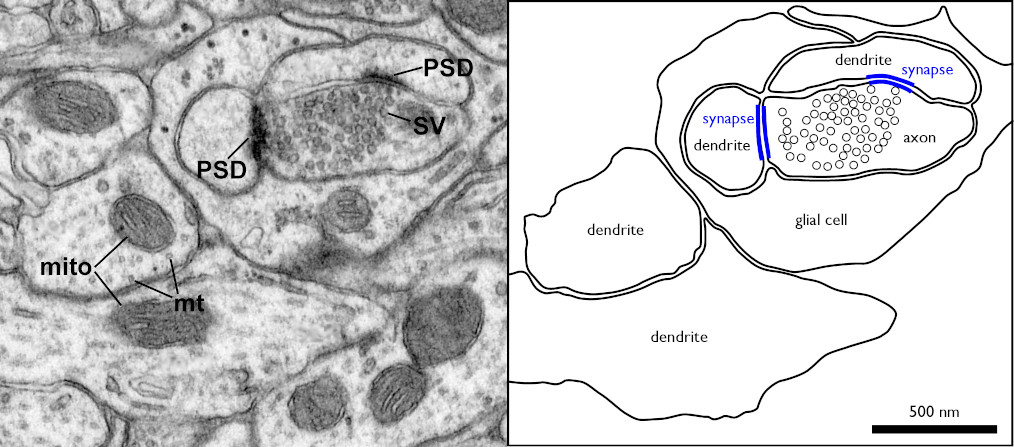
There are two kinds of synapses:
- Electrical synapses have gap junctions that allow ions to pass directly between the cytoplasm of the neuron and the cytoplasm of its target cell, letting changes in voltage pass through directly.
- Chemical synapses, by contrast, use an extracellular chemical signal. An axon’s ending at the synapse is packed with secretory vesicles. With each action potential, a few of the vesicles fuse with the plasma membrane to release an extracellular signalling molecule called a neurotransmitter into the synaptic cleft, the narrow space between the two cells. The neurotransmitter molecules bind to the extracellular portion of transmembrane proteins in the cell membrane of the target cell. These transmembrane proteins are called neurotransmitter receptors. Binding of a neurotransmitter molecule changes the shape of the neurotransmitter receptor, and this change in protein shape passes the signal into the target cell.
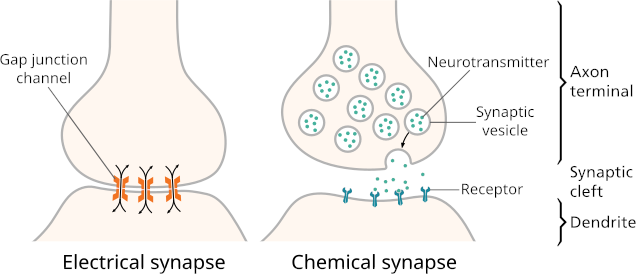
Two kinds of neurotransmitter receptor are common:
- Ligand[2] gated ion channels, and
- G protein coupled receptors.
Ligand-gated ion channels affect the target cell’s membrane potential directly by allowing specific ions to cross the cell’s plasma membrane. G protein coupled receptors signal through changes in the amount of a second small signalling molecule in the cytoplasm of the target cell. The intracellular, low molecular weight signalling molecules are called second messengers. The second messenger can open or close ion channels, or can have a wide range of other effects on the target cell. For more on cell surface receptors, see Section 3.7 and Chapter 4.
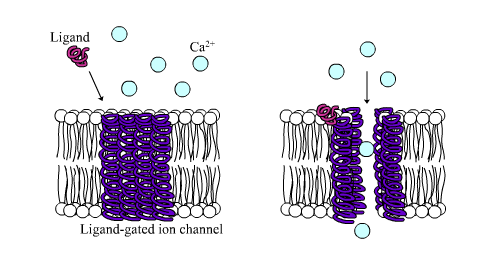
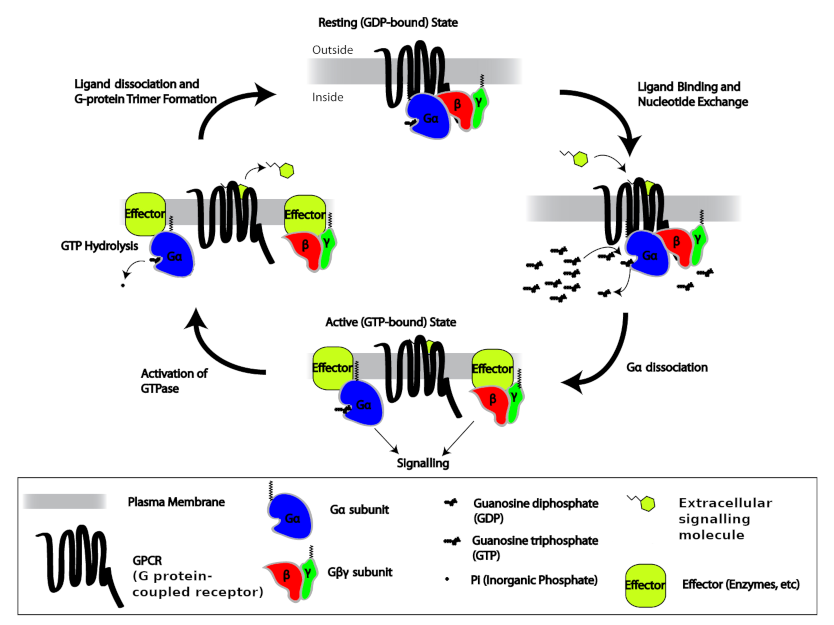
3.4 Neurotransmitters
Neurotransmitters are neuron-borne extracellular signalling molecules that bind to the receptor domain of a channel located in the membrane of the post-synaptic side of the synapse. Neurotransmitters are classified based on their chemical structure, and include:
- Acetylcholine, an ester of acetic acid and choline[3].
- The biogenic amines norepinephrine, epinephrine, histamine, serotonin and dopamine.
- The amino acids glutamate, aspartate, D-serine, γ-aminobutyric acid and glycine.
- Neuropeptides. Around one hundred different peptides, 3–100 amino acid residues long, act as neurotransmitters. The peptides substance-P and the enkephalins are examples of neuropeptides.
In addition, some neurons signal by making “gases” or “gasotransmitters”. These are very low molecular weight, uncharged intercellular signalling molecules that can readily diffuse through both lipid bilayers and water. They include nitric oxide, hydrogen sulphide, and carbon monoxide. These are not normally gases in the body: Even though these are gases when in pure form in the laboratory, the amounts produced in the body are far too low to create bubbles. Because the gasotransmitters can diffuse easily through the lipid bilayer of the cell membrane, the receptors for them can be inside the cell.
3.5 Humoral Signalling
Humoral communication relies on extracellular signalling molecules that bind to specific receptors on a target group of cells. These signalling molecules include hormones, growth factors, and some neurotransmitters. They are typically produced by ductless glands, termed endocrine glands (Greek: endon, inside; krinein, to distinguish; contrast with the word exocrine). Additionally, most living tissues produce some hormones and growth factors. Endocrinology is the study of chemical signals secreted into circulation within the body.
The word “hormone” comes from the Greek verb “hormô” meaning “to spur into action”. The word “hormone” was coined in response to the surprising discovery, in 1902, that the intestine can trigger digestive enzyme secretion by the pancreas without any nervous system input. One of the discoverers defined hormones as substances that “have to be carried from the organ where they are produced to the organ which they affect by means of the blood stream”. In more modern language: signalling molecules synthesized (made) by specialized cells that secrete and release them into the blood to exert specific biochemical actions on target cells located at distant sites.
With the discovery of growth factors and a wide range of other extracellular signalling molecules, the definition of endocrinology was extended to also include hormone-like molecules secreted locally and are mainly released into the interstitial space, exerting specific actions on target cells located nearby.
In vertebrate animals, glands that produce hormones and secrete them into the interstitial space or directly into the circulation include:
- Hypothalamus
- Pituitary
- Pineal
- Thyroid
- Parathyroid
- Adrenals
- Pancreatic Islets
- Gonads
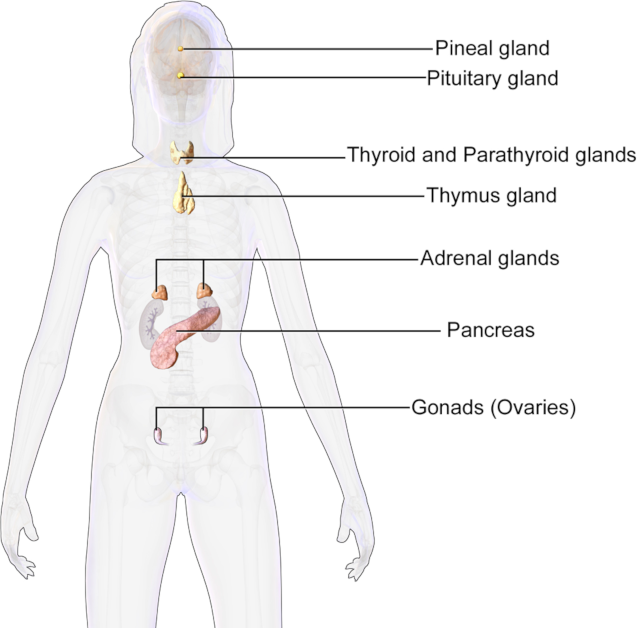
In addition, other organs with hormone-secreting cells can produce, convert, or secrete hormones or hormone-like substances, besides their primary functions. Examples in vertebrate animals include the brain, placenta, thymus, kidney, heart and the vascular system, skin, and adipose tissue. Overall, extracellular chemical signals function in a similar way regardless of their origin.
The main functional properties of the humoral signalling system are:
- does not initiate new functions, but regulate already established cellular processes;
- has relatively brief effects, and hormones fluctuate in concentration, responding to immediate physiological requirements. Albeit, in some cases hormones may cause permanent changes, such as sex differentiation, that persist even when the hormone is no longer required;
- has specific and sensitive effects that influence unique cellular responses at very low concentrations; and
- triggers a cascade of amplifying mechanisms meaning that a small amount of a chemical signal induces the release of a larger amount of another chemical signal and/or intracellular metabolites in the target cells until physiological demands are meet.
Once a signalling molecule reaches its target tissues, it binds to a specific receptor. Receptors are proteins with high affinity and high specificity for the signalling molecule. Thus, a receptor is able to discriminate between hundreds of other regulatory molecules that circulate in the blood stream and diffuse throughout the body’s tissues.
Affinity describes how well two objects stick together, while specificity describes how selective the binding is. Chewing gum may have high affinity for a lock, but the correct key has high specificity for the lock. The fewer ligands a protein can recognize and bind, the greater its specificity. For example, an antibody protein binds to only one specific antigen with high affinity, and has low affinity for all the other antigens, which are recognised by other antibodies.
The humoral signalling system can further ensure specificity by directly secreting chemical signals into confined areas, such as the interstitial spaces, or the synaptic clefts. In this way, bloodstream dilution is avoided, and effective concentrations of signalling molecules are established at the receptor level. Therefore, signalling molecules with affinity for widely distributed receptors are able to elicit specific responses within limited spaces without affecting other tissues.
Signalling Modes of Action. Many of the same signalling molecules that have systemic (whole body) effects also act more locally. This has inspired researchers to coin several additional terms based on the term “endocrine”. Here is a definition of “endocrine”, followed by the derived terms:
- Endocrine – the signalling molecule travels systemically through the bloodstream to target distant cells in the body.
- Paracrine – the cells that produce the signalling molecules are located in close proximity to the target cells.
- Neuroendocrine, occasionally called neurocrine – the extracellular signalling molecules are peptides or amines that are secreted by the neurons as either a neurotransmitter or a neurohormone.
- Juxtacrine – or contact-dependent signalling, refers to membrane-bound peptide molecules that bind to cell receptors located in close proximity, requiring physical contact between the cells involved.
- Autocrine – the chemical signal binds to receptors on the same cell that secretes the chemical signal.
- Intracrine – refers to the transformation of an inactive molecule into an active signalling form by enzymes in the target cell.
3.6 Extracellular Signalling Molecules
Extracellular signalling molecules can also be classified based on their chemical structure:
- Biogenic amines – derived from the amino acids tyrosine and tryptophan.
- Proteins and glycoproteins – water-soluble proteins, with structures ranging from short peptides to high molecular weight, dimeric glycoproteins.
- Steroids – cholesterol derivatives of low water solubility that are transported by protein carriers.
- Gases – nitric oxide, hydrogen sulphide and carbon monoxide.
Many extracellular signalling molecules dn’t fall into any of the categories listed above, since they can have a wide variety of chemical structures
Two other groups of chemical signalling molecules include the prostaglandins and retinoic acid. Prostaglandins are biologically active lipids, derived from the 20-carbon fatty acid arachidonic acid, generally known as eicosanoids, with hormone-like effects. They are autocrine or paracrine regulators that participate in a variety of endocrine mechanisms. Because prostaglandins are not produced at a specific site, but in many organs and cells in the animal body, they are described as general chemical signals and not as hormones. Retinoic acid is a metabolite of vitamin A required for growth and development. In chordates, retinoic acid is an intercellular signalling molecule generated in a specific region of the embryo and determines position along the anterior-posterior axis. Retinoic acid has several additional signalling functions later in embryonic development and in adult animals.
A common feature of all extracellular signalling molecules is their capacity of recognizing specific membrane or intracellular receptors with high affinity and specificity. Therefore, the definitions of extracellular signalling molecules also include the neurotransmitters that bind to membrane receptors; although the definition of neurotransmitters applies only to those signalling molecules with effects limited to the synapse.
3.7 Intracellular Signalling
Chemical signals transmit information into the cell through protein structures, termed receptors. Signalling molecules bind to receptors with high specificity, meaning that receptors can recognize a ligand among many different molecules that are usually present in the environment surrounding a target group of cells. Ligand-specific binding results in activation of intracellular reactions that amplify the signal. Large hydrophilic signalling molecules bind to receptors located on the cell membrane, while steroids and amino acid derivatives that are lipophilic cross the cells membrane and bind to intracellular receptors. Signalling receptors are classified into two main groups according to their location in the cell:
- cell surface receptors, located in the plasma membrane, and
- nuclear transcription factor receptors, located inside of the cell.
Cell Surface Receptors. Cell surface receptors bind hydrophilic molecules that cannot pass through membrane, including peptides and biogenic amines, which are poorly soluble in the lipids of the membrane. Ligand binding to membrane receptors can either alter the permeability of ion channels, or trigger a cascade of intracellular reaction that involve the synthesis of a second messenger system that activate intracellular proteins, usually enzymes, leading to a response. Cell surface receptors are usually glycoproteins with three defined structural and functional regions or domains:
- The extracellular domain – contains mostly hydrophilic amino acids that conform the ligand-binding site;
- The transmembrane domain – anchors the receptor into the plasma membrane by one or more stretches of approximately 20 to 23 hydrophobic amino acids; and
- The intracellular domain – contains mostly hydrophilic amino acids that are targets for phosphorylating enzymes that regulate activity of the receptor.
Cell surface receptors activate regulatory molecules called effectors that initiate a cascade of reactions that transform extracellular signals into intracellular signals. Effectors are either part of the membrane receptor itself, or a complex group of molecules associated with the receptor. Cell surface receptors can be classified into three major groups:
- G protein coupled receptors, also termed seven transmembrane receptors – include receptors for peptides, glycoproteins, neurotransmitters, and eicosanoids. These receptors are anchored into the plasma membrane by seven hydrophobic stretches of amino acids, with their amino terminal facing the extracellular space and the carboxyl terminal facing the cytoplasm. A distinct property of these receptors is that they are associated with a trimeric guanine nucleotide binding regulatory proteins, termed G-proteins, that function as molecular switches by binding guanosine-5′-triphosphate (GTP) and guanosine-5′-diphosphate inside the cell. G-proteins link the receptor with effectors, either an enzyme that makes an intracellular signal in the form a second messenger (see below), or an ion channel in the plasma membrane.
- Enzyme-linked receptors – proteins with a single transmembrane domain that anchors the molecule into the membrane. These proteins possess intrinsic enzymatic activity with an intracellular domain that contains the catalytic subunit of the enzyme. Most enzyme-linked receptors are protein kinases, meaning that they add phosphate to specific amino acid residues in specific target proteins. This enzymatic activity is called phosphorylation. For some receptors, the receptor protein itself is a target. When the receptor phosphorylates itself, the process is called autophosphorylation. Typically, kinase activity in the intracellular domain is activated by ligand binding to the extracellular domain. The receptor and the effector components of this signalling system reside within the same molecule. This group includes receptors for several hormones and growth factors.
- Ligand-gated ion channel receptors, also termed ionotropic receptors – ion-selective transmembrane channels formed by multi-subunit proteins. Ligand-gated ion channels open upon binding of specific ligands, such as the neurotransmitters acetylcholine (nicotinic cholinergic receptors), glutamate (ionotropic glutamate receptors), and serotonin (5-hydroxytryptamine; 5-HT receptors).
Cells at the receiving end of signals need to process the information internally. Signalling inside the cell is often by way of:
- low molecular weight chemical signals called second messengers;
- a cascade of protein phosphorylation; or
- regulation of gene transcription by transcription factors.
3.8 Second Messengers
For many receptors, activation by binding of a ligand results in an intracellular chemical signal mediated by a low molecular weight molecule. Most G protein coupled receptors function in this way. The low molecular weight intracellular signalling molecule is termed a second messenger, because in many cases it’s produced in response to an extracellular, hydrophilic “first” messenger molecule, such as a hormone or neurotransmitter that cannot cross the cell membrane. Well-known water-soluble second messengers include
- cyclic adenosine monophosphate (cAMP),
- calcium ions (Ca2+),
- cyclic guanosine monophosphate (cGMP), and
- inositol triphosphate (IP3).
Water-soluble second messengers bind to specific proteins that are either in the cytosol or exposed to the cytosol, turning their activity on or off. Some other second messengers are lipophilic, and are restricted to the cytosol-facing leaflet of cellular membranes. Examples of well-known lipophilic second messengers are diacylglycerol and the phosphatidylinositol phosphates. They bind to specific membrane proteins, turning their activity on or off.
3.9 Nuclear Transcription Factor Receptors
Nuclear transcription factor receptors bind lipophilic molecules that easily pass through the cell membrane, including steroids and thyroid hormones. This group of transcription factors comprises a large family of intracellular single subunit phosphoproteins that interact with membrane-soluble signalling molecules and directly affect gene transcription. They are also called ligand-activated zinc (Zn)-finger nuclear receptor transcription factors because they contain one atom of Zn that interacts via coordination bonds with four cysteine residues and recognize specific DNA consensus sequences in the regulatory regions of their target genes. Members of this family include the receptors for steroid hormones, thyroid hormones, retinoic acid, vitamin D and xenobiotic endocrine disruptors. Nuclear transcription factor receptors are exclusively found in metazoans, but must have appeared at early stages of animal evolution, because they are present in divergent animal groups such as sponges, ctenophores, and cnidarians. There are over 270 nuclear different nuclear transcription factor receptor genes in the nematode C. elegans, while humans have only 48.
Chapter 4 covers receptors and second messengers in a lot more detail.
3.10 Signalling Through the Senses
From the very early stages of evolution onwards, organisms were exposed to changes in their environment. Being able to detect and respond to a variety of physical and chemical signals is crucial for survival and reproduction. Accordingly, they developed different sensing mechanisms for detections of environmental signals as well as for transmission of information into their cells. Even the most primitive organisms receive and process light signals in the form of photons through their vision systems and chemical signals through their olfaction system.
Photoreceptors. The eyespots of unicellular organisms, the ocelli or eyes of invertebrates, and in the retina of vertebrates, have receptors with the capability of transforming light signals into a flow of ions into the cells. Light signals affect variables in the internal media of the animal body, such as the activity of some glands of internal secretion. The physiological reaction to the length of light is termed photoperiodism, defined as the developmental responses to relative length of light and darkness. A number of physiological changes depend on the photoperiod, including colour of fur and feathers, migration, hibernation, and sexual behaviour, among others.
Mechanoreceptors. Many animal species communicate by touch or by sound.
Chemoreceptors. The olfactory system also detects and conveys signals from the environment to the interior of the body. The olfactory system processes general odorants, such as small molecules originated from food, fire, or predators, and pheromones , which are molecules released from individuals of the same species that (Greek: phero, to bear or to carry) convey social or sexual cues. Chemoreceptors located on sensory hairs on the proboscis[4], legs, feet, and antennae of insects detect odours and other volatiles chemical signals for finding food and mates. Many insect species secrete sexual-attractant molecules into the air, from an abdominal gland, that are detected by chemosensory hairs on the antennae of the opposite sex, which enable the male to locate the female, or, in some species, the female to locate the male. Vertebrates including, deer, cow, sheep and dogs, among others, also produce volatile cues that convey sexual attraction signals.
Chemical signals are particularly important for communication between individuals is in most animal species. Pheromones – chemical signals between individuals of the same species – fall into the broader category of semiochemicals (Greek: semeion, signal). Chemical signals between individuals of differing species are sometimes called allomones (Greek: allos, other) or kairomones (Greek: kairos, opportune moment).
3.11 Summary
- Cells talk with each other by presenting or releasing specific extracellular chemical compounds;
- Cells use receptor proteins to detect those chemical signals;
- Receptors are cellular structures that recognize extracellular signalling molecules, such as hormones, growth factors, and neurotransmitters;
- Receptors are classified into two main groups:
-
- cell surface receptors, and
- nuclear transcription factor receptors;
- Tissues and organs integrate functions through a communication system that includes humoral, neural, and neurohumoral signals that activate intracellular mechanisms; Some of these regulate tissue-specific gene expression;
- Neural signals involve ion-driven voltage changes across the cell membrane;
- Ions cross the cell membrane through gated channels;
- There are two main kinds of gated ion channels:
-
- voltage-gated that open in response to changes in membrane voltage, and
- ligand-gated channels that open and close in response to ligand molecules, such as neurotransmitters;
- Cell communication systems include hormones and growth factors, secreted by one group of cells bind to specific receptors on a target group of cells;
- Hormones and growth factors are secreted into the interstitial space or directly into the circulation by ductless glands;
- Several humoral signalling modes can be defined, based on the relative location of the target cell relative to the signalling cell:
-
- endocrine,
- paracrine,
- juxtacrine, and
- autocrine;
- Neurons can release humoral signalling molecules; This is called neuroendocrine signalling.
3.12 Glossary
affinity –
allomone –
autocrine –
biogenic amine –
calcium ion –
cAMP – cyclic adenosine monophosphate
cell surface receptor –
cGMP – cyclic guanosine monophosphate
cyclic adenosine monophosphate –
cyclic guanosine monophosphate –
effector –
endocrine –
endocrine gland –
enzyme-linked receptor –
5-HT – 5-hydroxytryptamine also called serotonin
G protein coupled receptor –
gas –
glyocoprotein –
GPCR – G-protein-coupled receptor, also termed seven transmembrane segment receptor
GTP – guanosine triphosphate
hormone –
inositol triphosphate –
intracrine –
ionotropic receptor –
IP3 – inositol triphosphate
juxtacrine –
kairomone –
LGIC – Ligand-gated ion channel receptor or ionotropic receptor
ligand –
ligand-gated ion channel –
lipophilic –
metabotropic receptor –
neuroendocrine –
neuron –
neurohumoral –
neuropil –
neurotransmitter –
nuclear receptor –
odorant –
paracrine –
peptide –
pheromone –
phosphorylation –
photoperiodism –
prostaglandin –
protein –
protein kinase –
receptor –
second messenger –
semiochemical –
specificity –
synapse –
synaptic cleft –
transcription factor –
3.13 Suggested Readings
OpenStax College, Biology. OpenStax College. 21 June 2012.
http://cnx.org/content/col11448/latest/
Hirst BH. (2004). Secretin and the exposition of hormonal control. Journal of Physiology 15:339
https://www.ncbi.nlm.nih.gov/pmc/articles/PMC1665254/
Bayliss WM, Starling EH. (1902). The mechanism of pancreatic secretion. Journal of Physiology 28:325–352.
https://www.ncbi.nlm.nih.gov/pmc/articles/PMC1540572
Biology – Exploring the Diversity of Life (3rd Canadian Edition) by Russell et al., Nelson Education Ltd., 2016.
- Or near-contacts: As mentioned above, in Section 3.1, and below, in Figure 3.3, a chemical synapse has a narrow gap, called the synaptic cleft, between the two cells. ↵
- A ligand (Latin, ligare: to bind) is any kind of molecule, either natural or synthetic, that forms a complex with another molecule. In cellular signalling, the term refers to a molecule that produces a signal by binding to a site on a target protein resulting in a change of the protein’s shape (“conformation”). Ligands bind to target molecules by intermolecular forces, such as ionic bonds, hydrogen bonds, and Van der Waals forces in a reversible manner. ↵
- Phosphatidylcholine, another ester of choline, is an important component of biological membranes. Humans and many other animals are unable to make enough choline, so complete health requires choline in the diet. As a result, choline is sometimes considered to be a vitamin. ↵
- A proboscis is an elongated appendage from the head of animals. In invertebrates is a tubular extension of the mouth used for feeding and sucking. In vertebrates is an elongation of the nose of snout. ↵