2. The Nervous System
Chapter 2 – The Nervous System
- 2.1 Introduction
- 2.2 Cellular Composition of the Nervous System
- 2.3 Action Potentials
- 2.4 Nerves
- 2.5 Evolution of the Nervous System
- 2.6 The Central Nervous System
- 2.7 The Peripheral Nervous System
- 2.8 Summary
- 2.9 Glossary
2.1 Introduction
What’s the most complicated object in the universe? It isn’t in a distant star system billions of light years away. It’s sitting right on top of your shoulders, and weighs 1.4 kilos. It consists of 170 billion blobs of soft jelly. And there are 100 trillion connections between those blobs of jelly.
“We do have an organ for understanding and recognizing moral facts.”
– Canadian philosopher Paul Churchland
The object we’re talking about is the human brain, of course. Every song that has ever been composed, every novel that has ever been written, every mathematical proof that has ever been discovered, had its origin in the connections between cells in the human nervous system. This chapter discusses the cells and tissues that are specialized for processing and transmitting information.
2.2 Cellular Composition of the Nervous System
2.2.1 Neurons
Imagine the first, primitive animals more than 600 million years ago, barely more than colonies of undifferentiated cells. Suppose cells at one end of the animal’s body detect something in the environment that’s important for survival or reproduction – for example, food, or a mate… or a predator. How do those cells get the message to the cells at the opposite end of the body? If the body is small enough, and the message simple enough, a chemical or electrical signal can be relayed from cell to cell through all cells in the body, or a chemical signal can be circulated through the extracellular fluid of the animal’s body. But what if the body isn’t simple and small? This becomes impractical for urgent or complex messages (Chapter 3).
In the division of labour among types of differentiated cells, cells dedicated to sensing, processing, and transmitting information were among the first to evolve. Ideally, those cells would be optimized to overcome three engineering challenges:
- Carry information over a far greater distance than the normal diameter of a cell;
- Transmit the information over those distances much faster than can be achieved by passive diffusion of molecules or by flow of fluid; and
- Be able to encode a large amount of information.
What would such a specialized cell, called a neuron (Greek, neuron: nerve), look like?
It would have structures that allow it to receive information, and it would also have structures that allow it to send signals to other cells.
Most neurons have narrow, branched extensions of their cytoplasm, with surrounding cell membrane, which make contact with other neurons they receive information from. These projections are called dendrites (Greek, déndron: tree) because they often resemble branches of a tree. Dendrites carry electrical signals to the cell body of the neuron.
Most neurons have a single, long projection called the axon (Greek, axon: axle), that carries the neuron’s electrical signals away from the cell body to other cells. In almost all neurons, the axon comes in close contact with the cells that receive signals from the neuron – the exception being neurosecretory cells[1]. These physical contacts between neurons and their target cells are called synapses (Greek, synapsis: connection; from syn-: together, and haptein,: to join).
Thus, neurons can carry information over long distances – some axons in humans are close to one metre long, and up to twenty-five metres long in a blue whale. Neurons transmit information rapidly over those long distances using electrical signals. And neurons encode large amounts of information by being choosy which cells to connect to. We’ll explore these concepts in more detail in Chapter 5.
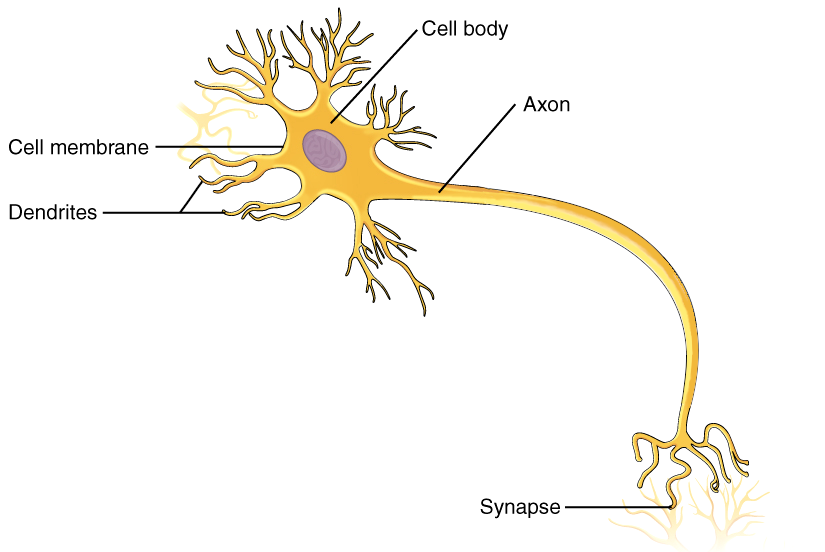
To sum up, the neuron is the basic functional unit of the nervous system. Neurons are highly specialized cells that can generate and transmit information in the form of electrical signals. Their structure is closely related to their functional role. Typically, a neuron is composed of three main parts:
- The cell body, or soma, contains the nucleus and most of the organelles.
- The axon is a long, slender projection that conducts signals from the neuron’s cell body. An axon arises from the soma of the neuron at a site called the axon hillock, which is a section of the neuron with large number of voltage-dependent sodium channels (Chapter 5). Axons are packed into bundles (“fascicles”) that in the peripheral nervous system form the nerves that transmit impulses to peripheral organs.
- Dendrites are branched projections of a neuron that receive electrochemical stimulation from other neural cells. Dendrites tend to be short compared with an axon.
The cell body may give rise to numerous dendrites, but never to more than one axon: Normally, the formation of one axon blocks the formation of any other axons from the same cell body.
While most neurons use their dendrites to pick up information from other neurons, many types of neuron pick up information from the extracellular fluid, from non-neuronal cells, or from the animal’s surroundings. Neurons that are specialized for receiving information from the environment are called sensory neurons . Many sensory neurons also have dendrites, but other sensory neurons have an unbranched projection called a cilium (Latin, cilium: eyelash) or a bundle of multiple unbranched projections called stereocilia. Depending on the type of sensory neuron, dendrites, cilia, or stereocilia can detect specific molecules, light, vibration, or pressure.
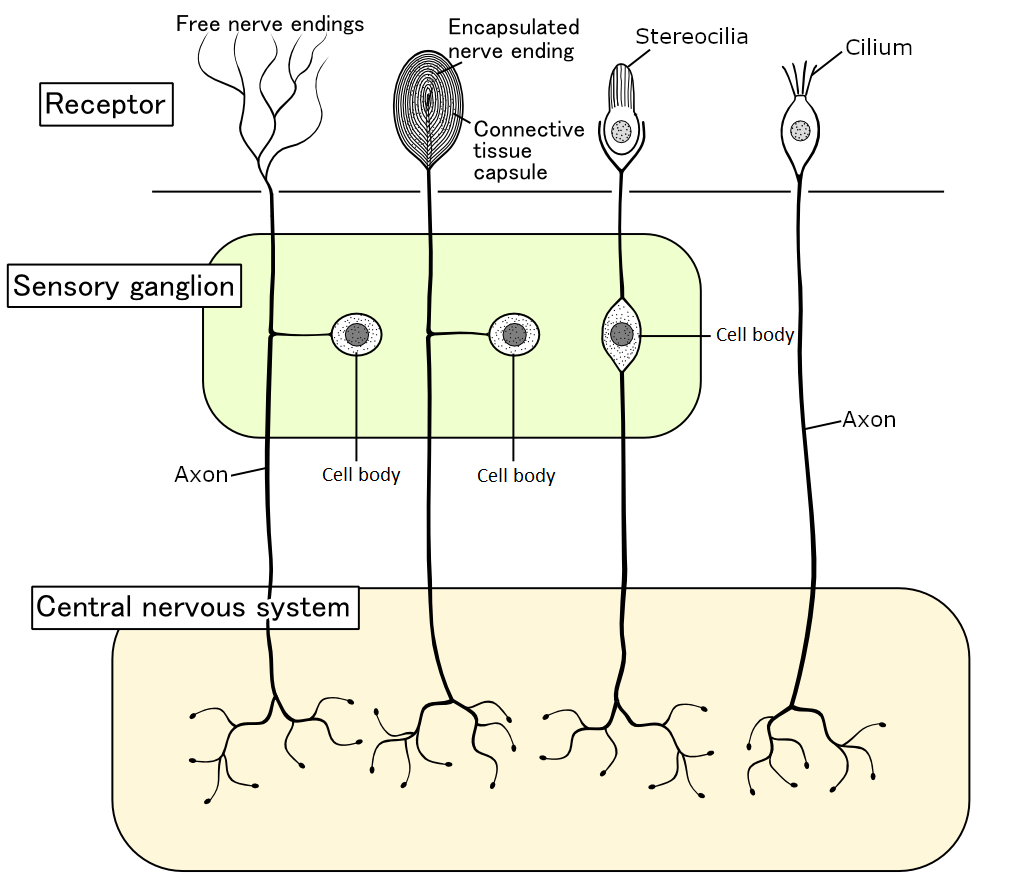
Neurons do not work in isolation: They are organized into circuits that process information. Information in a circuit flows through three types of neurons:
- Sensory neurons – are afferent neurons that detect information from outside the nervous system, and bring that information to other neurons;
- Motor neurons – are efferent neurons that send signals away from the central nervous system (CNS) to elicit a response, such as muscle contractions and secretion of glands;
- Interneurons – form interconnections between other neurons in the CNS. They tend to have many dendrites with axons typically short and highly branched, allowing forming complex connections with other cells. Interneurons are also called association neurons.
This chapter often refers to a central nervous system (CNS) and a peripheral nervous system (PNS). In many animals, nervous tissue is concentrated in one part of the body containing the CNS, but connects with the rest of the body through the PNS. Thus, we can define two main divisions of the nernous system in those animals. We’ll learn about the CNS and PNS in greater detail later in this chapter.
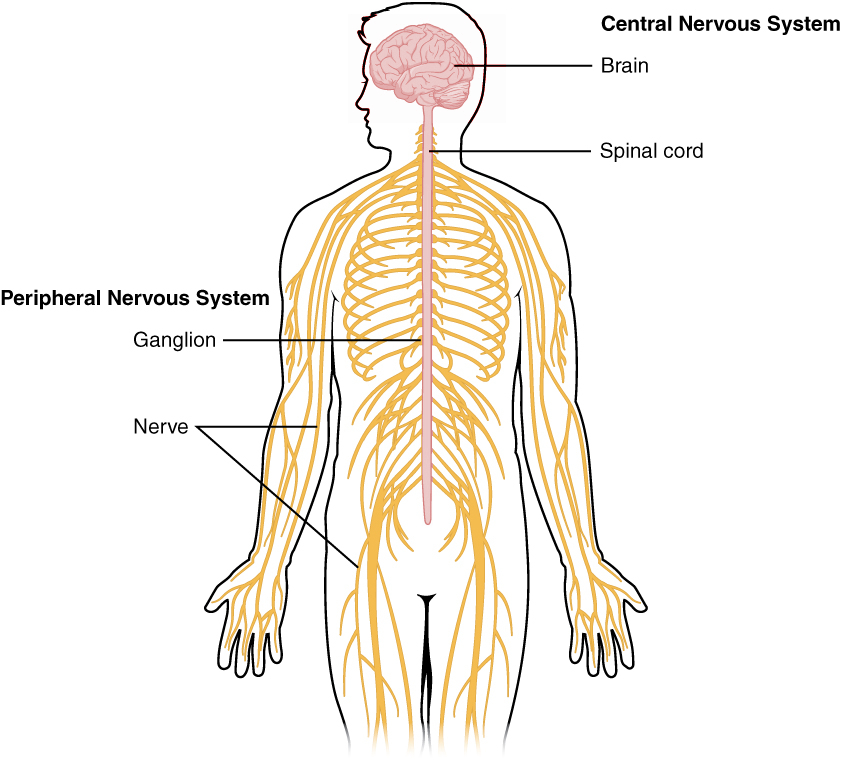
Two words that sound almost the same, but mean the opposite of each other:
afferent (Latin, afferens: bringing to; from ad: to, towards; : to bear, to carry), and
efferent (Latin, efferens: bringing out; from ex: out of)
We’ll be using the terms ‘afferent’ and ‘efferent’ a lot. To help remember them, we can relate them to English words that come from the same Latin roots:
Efferent axons exit the central nervous system to effect changes in the body.
Afferent axons add sensory inputs to affect the central nervous system.
In short, afferents are arriving fibres while efferents are exiting fibres. The last part of these two terms is related to the English words transfer, to bear, to bring, and even names such as Christopher.

Neurons come in a lot of different sizes and shapes. Neurons are classified into four main types based on their structure:
- Unipolar neurons – are neurons with only one extension from the cell body, an axon. When unipolar neurons have dendrites, those dendrites branch from the axon. True unipolar neurons are only found in invertebrates. In insects, for example, the brain contains many unipolar neurons, with their cell bodies near the periphery of the brain.
- Pseudounipolar neurons – are unipolar-like neurons characterized by an axon that emerges from the cell body and splits into two extensions that can reach a very long distance. One end of the axon branches into dendrites, while the other end of the axon forms synaptic connections with target neurons. The “pseudo” (Greek: “false” or “lying”) part of the name refers to the fact that the pseudounipolar neurons develop from bipolar neurons. In vertebrate animals, pseudounipolar cells are exclusively sensory: They transduce physical stimuli such as light, sound, and temperature into electrical signals transmitted by a single axon to the spinal cord and the brain.
- Bipolar neurons – are neurons with two extensions from the cell body: one axon and only one dendrite[2]. In some invertebrates, bipolar neurons are the most common type. Vertebrate animals, several kinds of bipolar neurons are specialized for transmission of senses, such as sight, smell, taste, hearing, and balance.
- Multipolar neurons – are neurons with a single (usually long) axon and many dendrites. They are the largest group of neurons in vertebrate animals, and are abundant in the cerebral cortex. Multipolar neurons include motor neurons and interneurons involved in integration with other types of neurons.

A fifth group of neurons includes those with no axon, termed anaxonic neurons, where the axon cannot be differentiated from the dendrites. Examples of anaxonic neurons can be found in the retina of the eye in mammals, in the part of the brain that processes the sense of smell in mammals, in the central nervous system of crayfish, and in the nerve net of cnidarians. Anaxonic neurons are rare compared with the other types.
2.2.2 Glial Cells
Neurons aren’t the only kind of cell that’s unique to the nervous system: In addition to neurons, the nervous system is composed of non-neuronal cells called glial cells or glia, originally seen as the “glue” of the nervous system (Greek, glia: “glue”). For example, the human brain contains roughly the same number of glial cells as neurons.
Most animals that have nervous systems have several different kinds of glia. Glia perform many different functions. Depending on the type of glia, glial cells provide nutrients to neurons, provide electrical insulation to axons, detect and transmit information to neighbouring neurons, guide neuron development and regeneration, engulf debris and help the immune system clear infections, maintain ion homeostasis, and help protect neurons in the brain from direct contact with most of the substances dissolved in the blood. Given all the different jobs that glia do in addition to physical support, maybe it’s unfortunate that the name “glia” (“glue”) has “stuck”!
Of the many kinds of glial cell that have been described in vertebrate animals, six types are relatively abundant and well-known:
- Astrocytes – (Greek, astron: star; cyte, cell) also known as astroglia, star-shaped glial cells that provide nutrients to the neurons and biochemical support to the endothelial cells that form the blood-brain barrier. The blood-brain barrier selectively blocks entry of most dissolved substances in the blood into brain tissue. Astrocytes maintain ion balance and are also involved in repair and scarring in the CNS.
- Microglia – form ~20% of the total glial cells. They are the macrophages of the brain and spinal cord and the main active immune defense of the CNS. Microglia scavenge damaged neurons, plaques, and other particles including infectious agents.
- Ependymal cells – form a thin epithelial membrane lining the large fluid-filled spaces (ventricular system) of the brain and the central canal of the spinal cord. The main function of the ependymal cells is the production and circulation of cerebrospinal fluid, a colorless fluid that protects the CNS. Choroid plexuses of the brain’s ventricles are special areas where ependymal cells make most of the cerebrospinal fluid.
- Oligodendrocytes (Greek, oligos: small; dendron: tree) – meaning cells with a few branches, form what is termed oligodendroglia, the tissue that electrically insulates axons in the CNS, mainly in the brain and spinal cord;
- Schwann cells – are the principal glial cells of the PNS. An outgrowth of the Schwann cells forms the electrically insulating myelin sheath that wraps around axons of motor and sensory neurons. Myelin sheaths are interrupted by the nodes of Ranvier, which are uninsulated gaps of ~1 micron formed between the myelin sheaths.
- Satellite cells – cover the cell bodies of neurons in the PNS. It’s unknown what satellite glial cells do for the neuron cell bodies they envelop, but there’s evidence they help the neuron maintain ion and neurotransmitter homeostasis, and supply nutrients to the neuron.
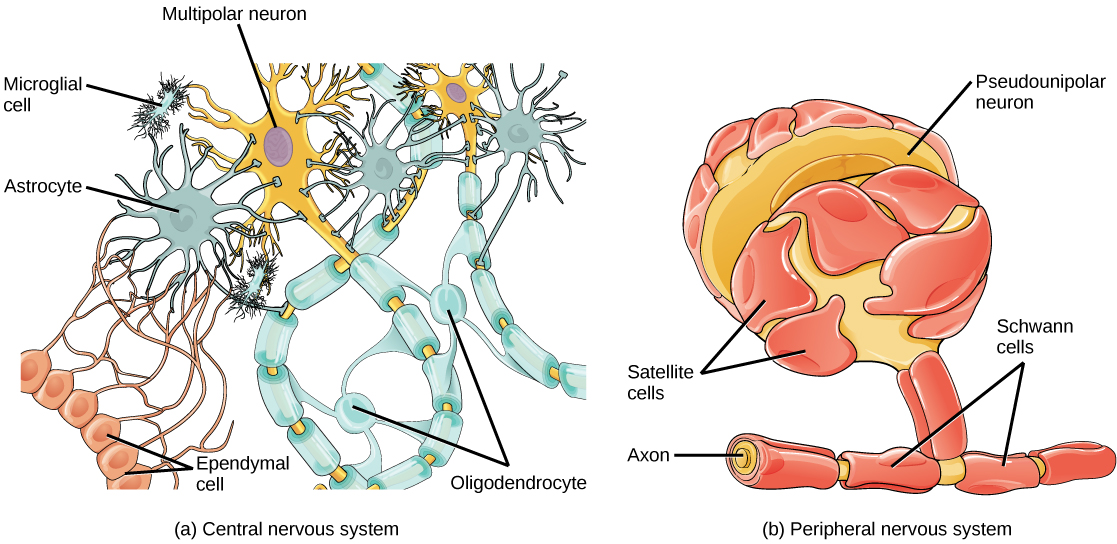
2.3 Action Potentials
As mentioned earlier, if a cell needs to send a signal quickly to a distant part of the body, electrical signals are the way to go. Electrical signals allow much more rapid transmission of information over long distances than can be achieved by passive diffusion of molecules or the circulation of extracellular fluid.
The nervous system is often likened to wiring in a machine. But there are a lot of important differences.
The lipid bilayer of the neuron cell membrane is an electrical insulator, because ions would have to break their electrostatic bonds with water to cross. However, the neuron’s cell membrane is embedded with ion channel proteins and ion pumps that make the cell electrically active.
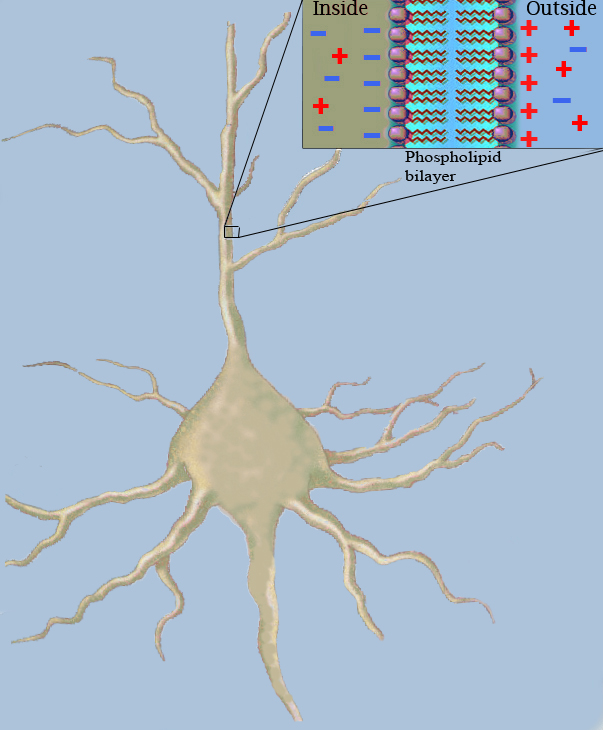
However, electrical signals are attenuated by the electrical resistance[3] of the axon’s cytoplasm and electrical capacitance[4] of the axon’s plasma membrane, both of which increase with axon length. As a result, most neurons of all but the tiniest animals are able to amplify electrical signals along the length of their axons to create action potentials – short-lasting changes in the membrane electrical charges that travel unidirectionally down the axon from the cell body.

Imagine a jellyfish gracefully pulsing as it swims in the water. We usually think of neurons generating action potentials in response to external signals, but many neurons generate action potentials spontaneousy (by themselves). In jellyfish, pacemaker neurons that spontaneously generate rhythmic potentials, which create rhythmic waves of muscle contraction that drive swimming. Nearly all complex animals have pacemaker cells, which are particularly important in brain and heart function.
Neurons aren’t the only type of cell that has action potentials. Muscle cells are a prominent example – muscle cells need to coordinate their contraction within the whole length of each cell and with each other, otherwise a muscle as a whole wouldn’t be able to contract. Inmost muscles, action potentials in muscle cells are what make this possible. Several other cell types have action potentials too, for example the hormone-secreting cells of the pancreas in mammals.
2.4 Nerves
Nerves are enclosed, cable-like bundles of myelinated axons that transmit signals in the peripheral nervous system. In nerves, three layers of connective tissue surround the axons. These three layers are called the endoneurium, the perineurium, and the epineurium, from the inside to the outside of the nerve. The axons with their myelin sheaths are embedded in the endoneurium. The perineurium bundles a group of axons. These bundles are called fascicles. The epineurium wraps fascicles together into a nerve. The central nervous system has analogous structures called nerve tracts, made of unmyelinated axons.
According to the direction of the signals that they conduct, nerves can be distinguished into three groups:
- Afferent nerves conduct signals from sensory neurons to the central nervous system.
- Efferent nerves conduct signals from the central nervous system along motor neurons to their target muscles and glands.
- Mixed nerves contain both afferent and efferent axons, and thus conduct both incoming sensory information and outgoing muscle commands in the same bundle.
Anatomically, nerves in vertebrate animals are classified into two groups, the cranial nerves and the spinal nerves.
Cranial nerves emerge directly from the brain, mostly from the brainstem. They innervate the head and neck and parts of the body. They emerge in pairs, which means that are present on both sides of the body. Those nerves with paths within the skull are termed intracranial nerves, while those that emerge through holes in the skull (foramina) are termed extracranial nerves. Cranial nerves have descriptive names, although classically are numbered with Roman numerals from I to XII, based on the order in which they, front to back, emerge from the brain:
- – Olfactory
- – Optic
- – Oculomotor
- – Trochlear
- – Trigeminal
- – Abducens
- – Facial
- – Vestibulocochlear
- – Glossopharyngeal
- – Vagus
- – Accessory
- – Hypoglossal
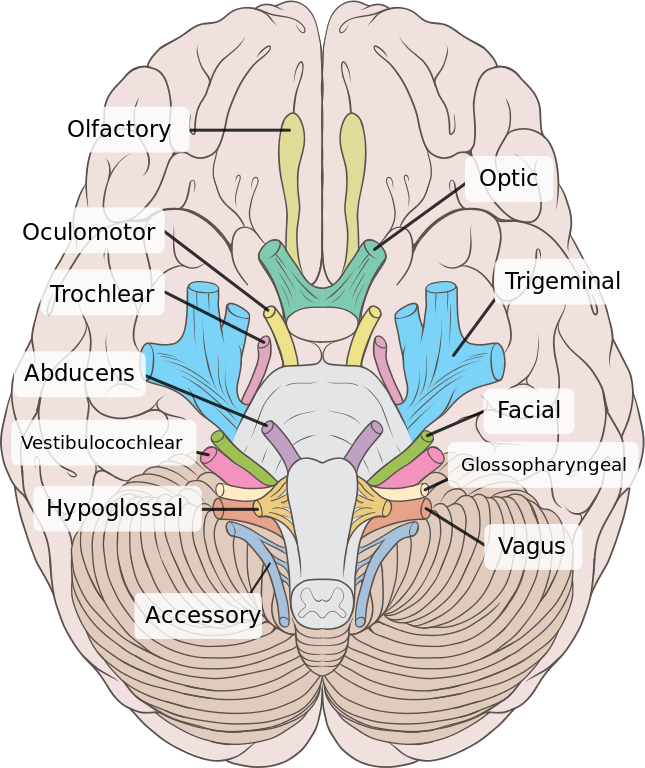
The vagus nerve (Latin: vagus, “rambling, wandering”, cranial nerve X, was called the “the wanderer” by early anatomists because it wanders a long distance among the internal organs of the body cavity. Most of the afferent axons bringing sensory information from the internal organs of the thorax and much of the abdomen run through the vagus nerve, and likewise, most of the efferent axons sending information to the same internal organs run through the vagus. Because the vagus nerve serves so many of the internal organs, it’s enormously important to the physiology of vertebrate animals. You’ll be hearing a lot more about the vagus nerve in later chapters of this book. Like the other cranial nerves, the vagus nerve is actually a pair of nerves, left and right.
In addition, a thirteenth cranial nerve or terminal nerve, sometimes termed nerve O or N, has been described, which is very small in humans. The olfactory (I) and optic (II) nerves originated in forebrain are considered part of the central nervous system (CNS), while the rest are considered components of the peripheral nervous system (PNS).
Fishes and amphibians have a slightly different set of cranial nerves.
Spinal nerves innervate much of the animal body through the spinal cord. They are formed by combination of fibres from the posterior and anterior roots of the spinal cord. The posterior root carries afferent sensory signals to the brain, while the anterior root carries efferent motor signals from the brain. There are 31 pairs of spinal nerves in the human body, one on each side of the vertebral column. They emerge through the intervertebral openings between adjacent vertebrae, and are identified according to the corresponding vertebra of the spinal column.
Thus, they are grouped into pairs:
- Eight cervical,
- Twelve thoracic,
- Five lumbar,
- Five sacral, and
- One coccygeal.
Thus, there are 43 pairs of nerves emerging from the CNS of the human body, and each nerve contains a sensory section and a motor section.
2.5 Evolution of the Nervous System
The first animals to evolve neurons would have presumably had a diffuse network of neurons without centralization, the simplest way to organize a nervous system. Modern animal phyla with such simple nervous systems include the diploblastic phyla Ctenophora (Greek, kteis: comb, and pherō: carry), for example the comb jellies, and Cnidaria (Greek, cnidos: stinging nettle), for example the sea anemones, hydras, jellyfish, and corals.
Differences at the molecular level have led some researchers to speculate that the nervous system originated at least twice in evolution, independently in the ancestors of the ctenophores and in the ancestors of all other eumetazoa, including cnidarians. Nevertheless, both the ctenophores and the cnidarians have nervous systems that are more complex and advanced than the first animals to have nervous systems. For example, both have sensory organs. Comb jellies and jellyfish have statocysts, sac-like sensory organs that detect the direction of gravity and acceleration. Jellyfish often have ocelli, sensory organs that detect light. The box jellyfish even have true eyes, with a lens to focus light and a retina, a sheet of light-sensing cells that detect images.
Additionally, sophisticated neural integration may exist in cnidarians. Motion in cnidarians is controlled by motor neurons that connect to muscle cells through synapses[5]. While the axons of the sensory neurons sometimes make direct connections to motor neurons, in other cases an intermediate interneuron participates, integrating information.
Cnidarians use longitudinal muscles to shorten the body stalk, and circular muscles to narrow the body cavity. This combined activity causes the stalk to lengthen and stimulate simple movements of the body wall and tentacles. In jellyfish, the network of neurons controls the body wall to contract and expand for swimming.
The type of muscle of ctenophores is similar to the muscle of mesodermic origin of more complex animals. Thus, some authors classify ctenophores as triploblastic rather than diploblastic.
For an animal that is highly active, the faster it can process complex information, the better it will be able to survive and reproduce. In order to process complex information the most rapidly and efficiently, neuron cell bodies need to be close to each other. Clusters of neuron cell bodies with localized interconnections are termed ganglia (singular: ganglion). Ganglia are seen in jellyfish and in all of the more complex eumetazoa, consistent with an evolutionary advantage for grouping nerve cell bodies together.
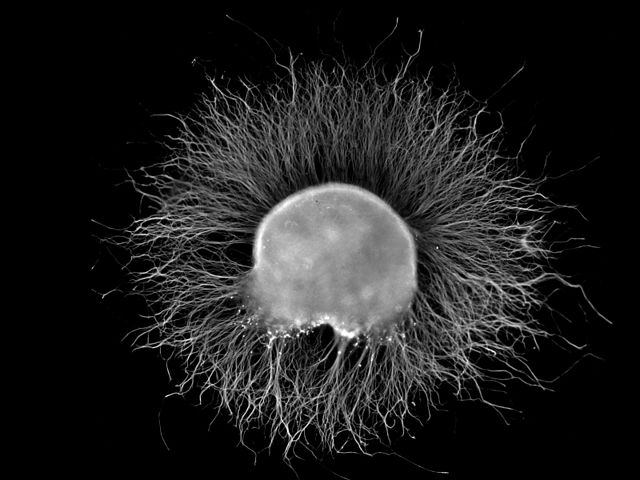
Cnidarians are radially symmetrical. How are nervous systems organized in bilaterally symmetrical animals? Unlike radially symmetrical animals, bilaterally symmetrical animals have a front (anterior) and a rear (posterior) end. The front end encounters new environments first, therefore concentrating sensory organs in the front end would be evolutionary advantageous for rapid processing of sensory inputs.
Platyhelminthes (flatworms), which like all bilaterians are triploblastic, have a centralized nervous system composed of a pair of cerebral ganglia, with longitudinal nerve cords that coordinate nervous activity, in conjunction with a peripheral nervous system of transverse nerves that form ventral surface nerve nets. The anterior location of sensory organs like the light sensitive eyespot, chemosensitive cells and sensory cells are concentrated in an organ called auricles (Latin, auris: ear), which allow rapid integration of sensory information and therefore respond with reactions that are more complex. In the more complex animals, the anterior ganglia evolved to form a brain, which can be considered a pair of “super ganglia”.
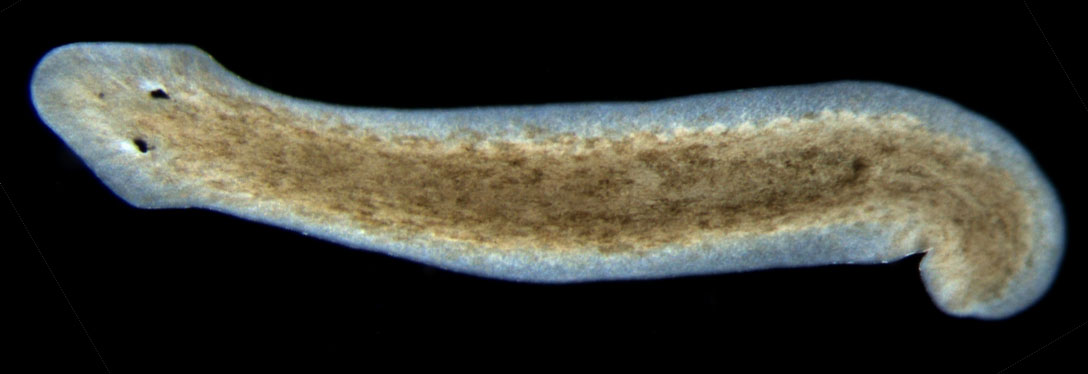

Arthropods, including insects, arachnids, myriapods, and crustaceans, have a high degree of encephalization. They have a brain consisting of dorsal and ventral pairs of ganglia and major sensory structures mainly consisting of eyes and antennae. A ventral nerve cord enlarges into a pair of ganglia in each body segment. In many insects, especially in adults, these ganglia are fused into larger masses that form secondary control centres.
Despite their tiny brains compared with ours, honeybees and monarch butterflies accomplish astonishing feats of navigation.
The most advanced brains are found in cephalopods and vertebrates. The cephalopod Octopus (Greek, okto: eight, pous: foot) has the most pronounced intelligence of the known invertebrates. Octopuses have maze-running and problem-solving skills that rival the abilities of mammals. However, it is a statistical fallacy to conclude that octopuses can accurately predict World Cup soccer matches, the FIFA 2010 performance of Paul the Octopus notwithstanding. Octopuses have a complex, lobed brain with clearly defined motor and sensory regions, for example optic lobes linked to large, complex eyes. Nevertheless, the nervous system of octopuses is less centralized than that of vertebrates. The ganglia of the octopus’ eight arms make local decisions independently of the brain’s awareness. In contrast, the brain handles a higher level of abstraction, such as how to get from one’s home aquarium tank to the tank down the hall with the tasty clams, and back without any humans noticing.
The vertebrates show the greatest amount of encephalization of any animal group. In vertebrates, the nervous system consists of the central nervous system (CNS) with a large brain and spinal cord, and the peripheral nervous system (PNS), which includes all neurons and neuron projections located outside of the CNS. The second half of this chapter describes the anatomy and function of the vertebrate nervous system in greater detail.
A complex body does not necessarily require a centralized nervous system. The echinoderm starfish (sea star) is a highly complex marine invertebrate, which like the cnidarians has radial symmetry. Sea stars have a nerve ring around the mouth. This nerve ring connects to radial nerves extending into the arms allowing the mouth and arms to operate independently. Starfish have many tiny sensory organs, and are sensitive to touch, light, temperature, orientation and chemicals. They have eye spots located at the end of the arms composed of simple ocelli with pigmented cells that respond to light. Despite the complete lack of encephalization, sea stars are capable of higher-level learning, seemingly purposeful behaviour, and co-ordination among the arms eerily reminiscent of highly encephalized bilaterians.
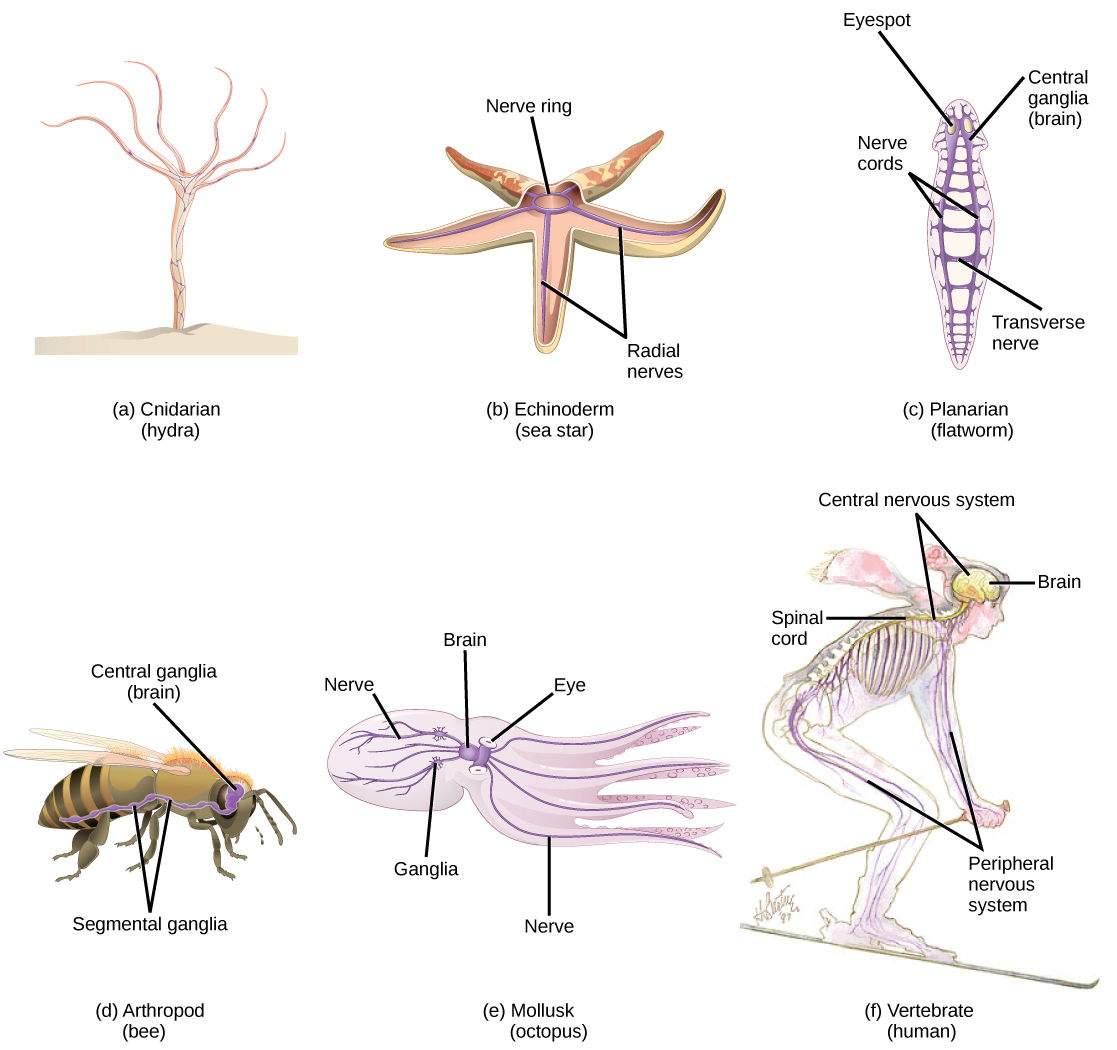
2.6 The Central Nervous System
At early stages of embryo development, the CNS is composed of a simple neural plate that bends and folds to form a groove termed the neural tube. The anterior end of the neural tube develops into the brain and the posterior portion develops into the spinal cord. The neural tube forms a central hollow that becomes the ventricles of the brain, and a central canal that follows along the spinal cord. The ventricles of the brain are cavities filled with cerebrospinal fluid that acts as a cushion or buffer, providing mechanical and immunological protection to the brain inside the skull. Three basic divisions can be observed in the developed brain: hindbrain, midbrain, and forebrain.
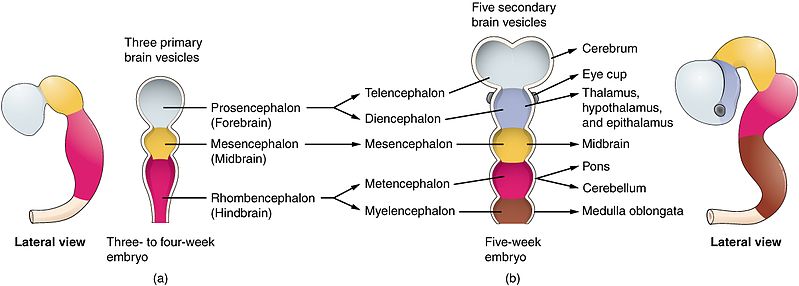
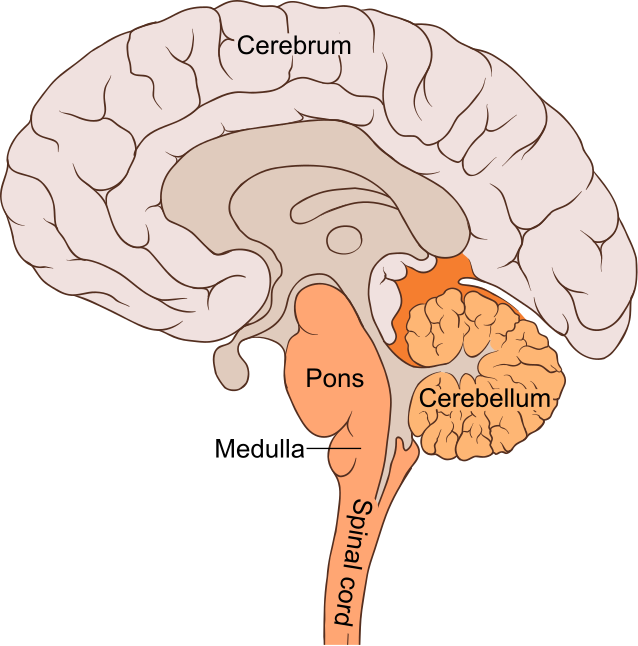
The Hindbrain – subdivides into the myelencephalon and the metencephalon:
- The myelencephalon gives rise to the medulla oblongata that connects the higher levels of the brain to the spinal cord. The medulla oblongata coordinates several functions of the PNS, including respiration, cardiovascular regulation, vomiting, coughing, sneezing and swallowing.
- The metencephalon gives rise to the cerebellum and pons. The cerebellum is involved in motor control of the body by coordinating movements with precise and accurate timing. It receives inputs from the body sensory systems through the spinal cord and other regions of the brain. The pons is a major traffic centre for information passing between the cerebellum and the telencepahlon.
The Midbrain – does not subdivide and it is termed the mesencephalon that processes several types of sensory inputs, including vision, hearing, smell and arousal[6].
The Forebrain – subdivides into the diencephalon and the telencephalon:
- The diencephalon gives rise to posterior forebrain structures, including the thalamus, hypothalamus, the posterior part of the pituitary gland (neurohypophysis) and pineal gland:
- The thalamus is located beneath the cerebrum and is the centre that receives sensory input and relays it to the regions of the cerebral cortex involved in motor responses;
- The hypothalamus is also located beneath the cerebrum and is the primary centre for homeostatic control of the internal media;
- The neurohypophysis – part of the pituitary gland – is a large projection of axons from hypothalamic neurons that terminate behind the anterior pituitary gland. The neurohypophisis stores and releases neurohormones;
- The pineal gland is a small endocrine gland that produces the serotonin-derived hormone melatonin, which is involved in modulation of sleep patterns and seasonal and circadian rhythms.
The telencephalon is the embryonic structure that gives origin to the cerebrum, which is divided into two symmetric hemispheres, the right and left hemispheres. A major neural tract called the corpus callosum connects both hemispheres. The cerebrum controls higher functions such as thought, memory, language, and emotions as well as voluntary actions in the body. The cerebrum consists of the basal nuclei, limbic system, and cerebral cortex:
- The basal nuclei – also called the basal ganglia, are a group of nuclei that surrounds the thalamus on both sides and lies beneath the cerebral cortex. The basal nuclei are involved in planning and learning movements and function via a complex circuitry to initiate or inhibit movements.
- The limbic system – refers to certain areas of the telencephalon and parts of the diencephalon. It is made of evolutionarily older structures that form an inner layer at the base of the forebrain. These structures include the olfactory bulb – which processes information about smells received by the olfactory system; the amygdala – which is involved in understanding and remembering emotions; the hippocampus – composed of several layers of cells that are connected in a circuit establishing memories for spatial locations, facts, and sequences of events. The hippocampus also receives extensive inputs from the olfactory bulbs. Signalling to the hippocampus triggers memory and is used to sense smell as a way of learning and remembering environments.
- The cerebral cortex – is the brain’s outer layer of neural tissue. The main component of the cerebral cortex is the gray matter that consists predominantly of neural cell bodies, glial cells, synapses and capillaries.
In the central nervous system of mammals, glial cells, together with the capillaries and neurons, form a grey-brown coloured tissue called grey matter. Glial cells and capillaries maintain stability of the nervous system tissue, provide support and protection to the neurons and play a role in neurotransmission. In the human brain, there are about three glial cells for every two neurons in the cerebral gray matter.
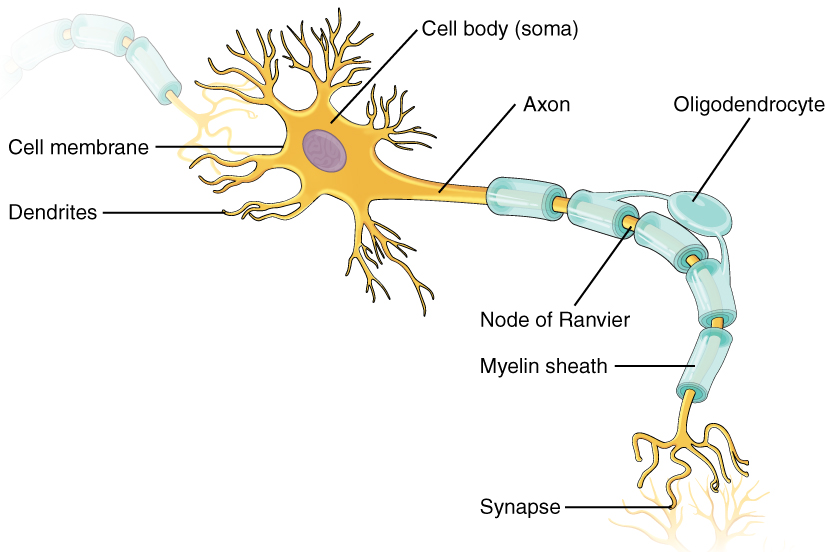
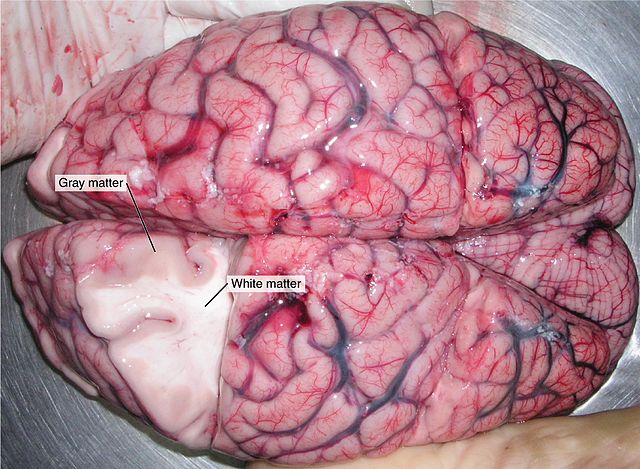

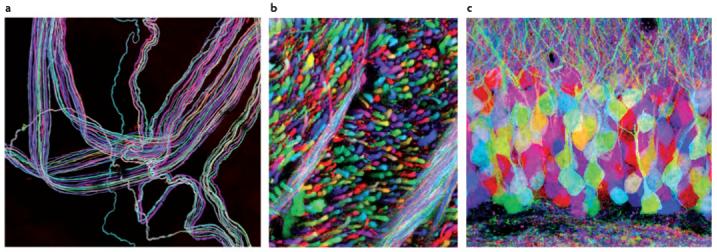
Non-neural cells and a white insulating material produced by some glial cells, called myelin, cover axons, constituting the white matter of the tissue of the CNS, mainly formed by myelinated axons.
The most recent development of the nervous system in mammals is the neocortex – smooth in rodents and other small mammals, while larger animals with larger brains show extensive folding of the entire cortex to fit the larger number of neurons. Overall, the cerebral cortex plays a key role in memory, attention, perception, awareness, thought, language and consciousness. In primates and other larger mammals, the neocortex folds into deep grooves. Folding of the neocortex allows the surface area of to increase beyond what could otherwise fit in the same size skull. Thus, the total mass of the brain relative to the size of the animal increased, permitting to undertake tasks that are more complex.
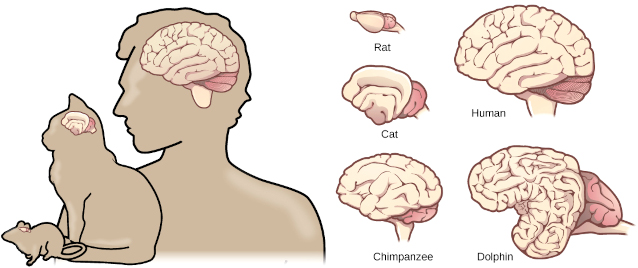
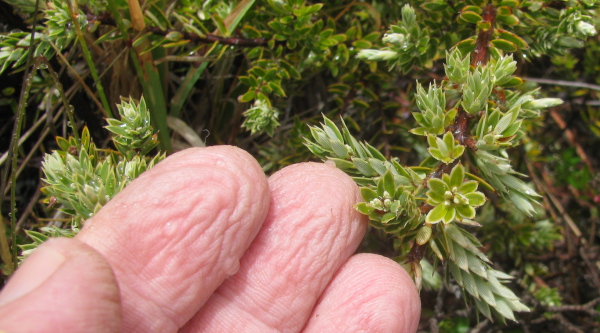
The skull protects the brain located in the cranial cavity, while the vertebrae protect the spinal cord. Both, organs are enclosed in the meninges, a system of membranes that envelops the CNS. In mammals, the meninges are composed of three layers of solid tissue:
- The dura mater (Latin, dura: hard, mater: mother) is a thick membrane external membrane that covers the brain and spinal cord;
- The arachnoid mater (Greek, arakhnē: spider) is a membrane attached to the inside of the dura mater surrounding the brain and the spinal cord;
- The pia mater (Latin, pia: tender) is a thin, translucent, mesh-like meningeal membrane firmly attached to the brain tissue and loosely connected to the arachnoid mater. The pia mater covers nearly the entire surface of the brain.
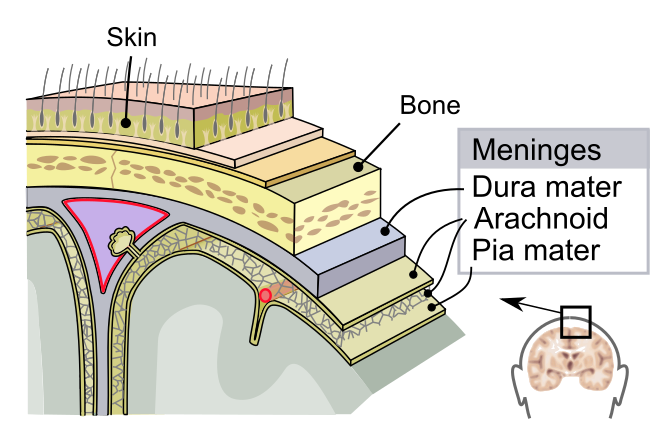
The primary function of the meninges and the cerebrospinal fluid is to provide mechanical and immunological protection to the CNS. The brain’s extracellular fluid and the circulating blood are separated by the blood-brain barrier, which is a complex structure of tight junctions around the capillaries, exclusive to the nervous system that do not exist in the rest of the circulatory system. The blood-brain barrier restricts the diffusion of microscopic materials, such as bacteria and large or hydrophilic molecules into the cerebrospinal fluid, while allowing the diffusion of small hydrophobic molecules (O2, CO2 and some hormones) and actively transport metabolic products such as glucose that are essential for the normal functioning of the CNS.
The spinal cord is a long, thin, tubular bundle of nervous tissue that transmits neural signals between the brain and the rest of the body through the medulla oblongata (Latin, oblongo: long). It extends from the occipital bones of the skull to two thirds of the vertebral column. The spinal cord is divided into five regions, cervical, thoracic, lumbar, sacral and coccygeal. From the human spinal cord emerge 31 pairs of mixed – motor and sensory – nerves.
2.7 The Peripheral Nervous System
The PNS consists of all the nerves outside of the brain and spinal cord. These nerves are not protected by bones or by the blood–brain barrier. The PNS is divided into the somatic nervous system (SNS) – including the motor and the sensory systems – and the autonomic nervous system (ANS). The functions of the PNS are to:
- Connect the CNS to the limbs and organs;
- Conduct motor information, which travels down;
- Conduct sensory information in the reverse direction, and
- Coordinate certain reflexes.
The spinal nerves are named based on where they connect to the spine. The cervical spinal nerves emerge from the spinal cord in the region of the neck and innervate the head, neck, arms, hands, and diaphragm. The thoracic spinal nerves emerge from the spinal cord in the chest region, and innervate the intercostal muscles (involved in breathing) and the heart. The lumbar, sacral, and coccygeal spinal nerves emerge in the lower back and pelvis and innervate the legs, pelvis, bladder and bowel. Although the spinal nerves emerge from the vertebral column along its entire length, the spinal cord itself does not reach all the way down into the lumbar region. Instead, the lumbar, sacral and coccygeal nerves branch out from the spinal cord and travel down the vertebral column to the point where they exit. Thus, the bottom third of the vertebral column contains spinal nerves but not spinal cord. The spinal cord also contains neural circuits that can independently control reflexes.
Axons emerging from the brain or the spinal cord first connect to dendrites that are located in ganglia that provide relay points between the CNS and the PNS, and with the target organs. Ganglia also interconnect with other ganglia to form a complex system of ganglia.
There are two main groups of ganglia in the PNS:
- Dorsal root ganglia (also known as the spinal ganglia) contain the neuron’s bodies of sensory (afferent) nerves;
- Autonomic ganglia contain the neuron bodies of autonomic nerves. In the autonomic nervous system, fibres from the central nervous system to the ganglia are known as preganglionic fibres, while those from the ganglia to the effector organ are called postganglionic fibres.

2.7.1 The Somatic Nervous System
Thus, the somatic nervous system is part of the PNS. It consists of cranial and spinal nerves that carry sensory information into the CNS and motor commands out of the spinal cord. Cranial nerves carry sense signals from the odour receptors of the nose, the light-detecting cells of the eye, the eye muscles, the mouth, taste, ear, neck, shoulders, and tongue. Spinal nerves carry sensory information into and motor commands out of the spinal cord. Motor neurons of efferent nerves control all voluntary muscular systems within the body, responsible for stimulating skeletal muscle contraction. Interneurons integrate sensory input and motor outputs in the somatic nervous system.
The Somatic Involuntary Reflex Arcs. In addition to controlling the activity of voluntary muscles, the somatic nervous system is involved in involuntary movements known as reflex arcs. The reflex arc is a neural circuit composed of only two neurons that synapse in the spinal cord and directly links a sensory input with a specific motor output. Thus, the reflex arc creates an automatic link between sensory and motor neurons that permit a rapid protective response to an injury by directly activating spinal motor neurons without the delay of routing signals through the brain. The brain will receive sensory input while the reflex is carried out, but the analysis of the signal takes place after action occurs. The patellar reflex is a functional clinical test of the lumbar 2, 3 and 4 segments of the spinal cord.
2.7.2 The Autonomic Nervous System
The autonomic nervous system (ANS), also termed the visceral nervous system, controls most of the involuntary visceral functions, those that are below the level of consciousness, including heart rate, digestion, respiration rate, salivation, perspiration, diameter of the pupils, urination, and sexual arousal. Some functions, such as breathing, work in coordination with consciousness.
The efferent nerves of the ANS are further divided into two divisions, the sympathetic division and the parasympathetic division. Both divisions act on the same organs with opposing actions, but not always necessarily, because they also cooperate in regulating some functions. The functions of the ANS are integrated by the hypothalamus that receives regulatory signals from other parts of the brain.
The classical description of the ANS is that the sympathetic division is a rapid mobilization system involved in rapid reactions like fight-or-flight, while the parasympathetic division is a slow activated system mostly affecting rest. The exception to this generalization is sexual arousal and orgasm, where both the sympathetic and the parasympathetic divisions are involved.
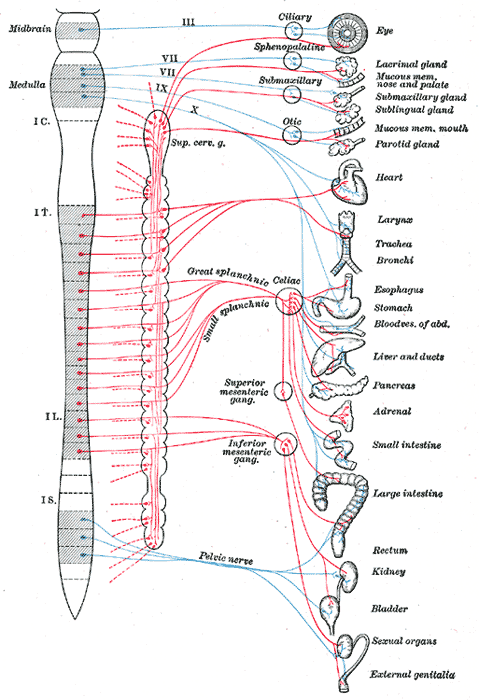
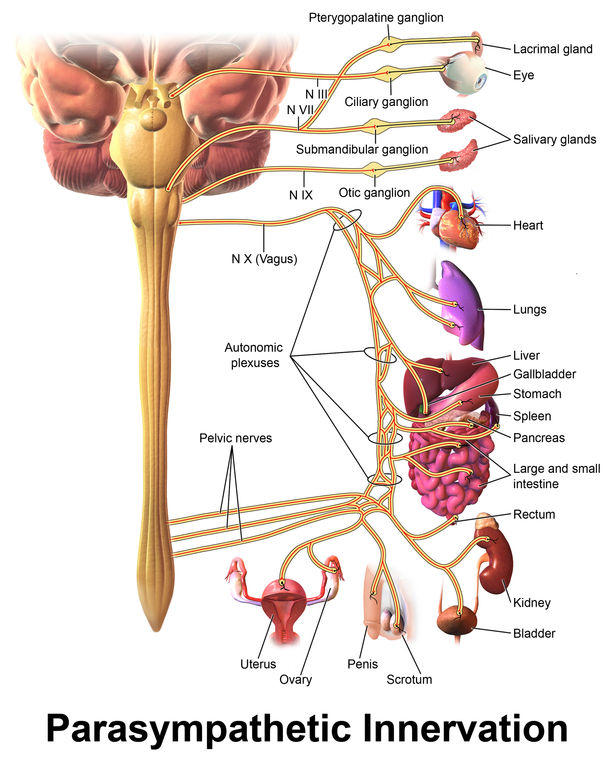
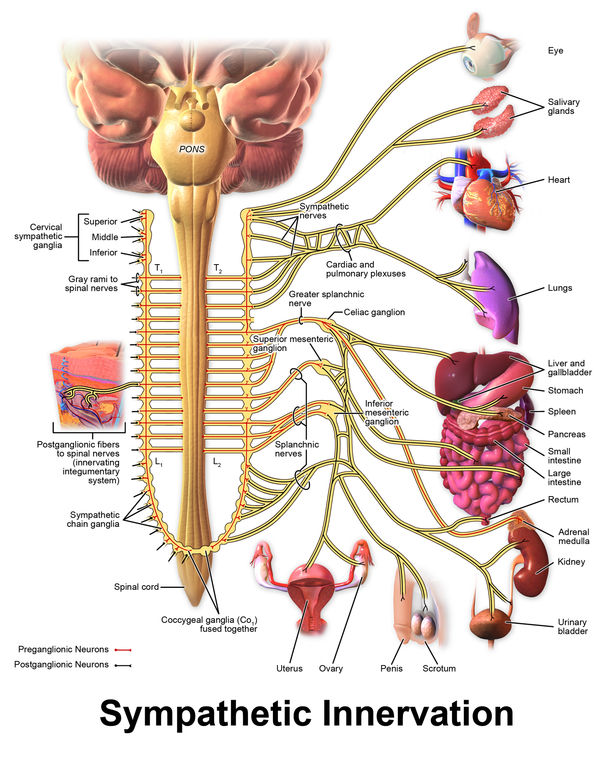
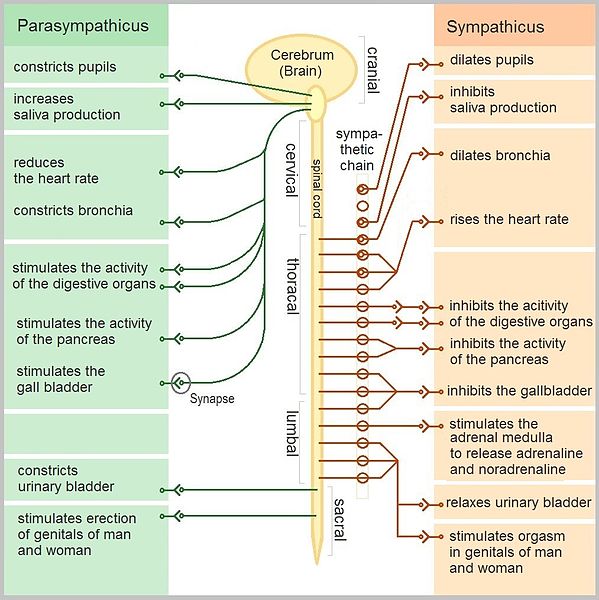

The Parasympathetic Division of the ANS – The main nerves of the parasympathetic nervous system are the third, seventh, ninth, and tenth (also termed vagus nerve) cranial nerves, which originate in the medulla oblongata; and the second, third, and fourth sacral nerves, termed the pelvic splanchnic nerves. Because of these locations the parasympathetic are termed craniosacral nerves.
The main anatomical feature of the parasympathetic nerves is that each preganglionic neuron synapses with just a few postganglionic neurons located near or in the effector organ. The functions of the parasympathetic nervous system can be summarized as follows:
- promotes the “rest and digest” response by returning organs to regular function;
- responds to the greater metabolic demands during and after digestion by dilating blood vessels of the gastrointestinal tract and increasing blood flow following consumption of food;
- in coordination with digestive functions, stimulates salivary gland secretion, accelerates peristalsis, mediates digestion and facilitate absorption of nutrients;
- constricts the bronchiolar diameter when demand for oxygen is reduced;
- reduces heart rate;
- in the eyes, constricts the pupil, reducing the amount of light getting into the eye, and constricts the ciliary muscle, bulging the lens to focus on closer objects; and
- stimulates sexual arousal and cause erection of the genitals.
A useful acronym to summarize the functions of the parasympathetic nervous system is SLUDDSA, standing for Salivation, Lachrimation, Urination, Digestion, Defecation, and Sexual Arousal.
The Sympathetic Division of the ANS – consists of cell bodies of the lateral horn of the spinal cord axons emerging from the first thoracic to the second lumbar nerves. Because of these locations, the sympathetic are termed thoracolumbar nerves. Fibres of the sympathetic nervous system innervate tissues in almost every organ. The salient anatomical feature of the sympathetic nerves is that each preganglionic neuron synapses with postganglionic neurons located far from the effector organ.
The preganglionic neurons of the sympathetic nervous system synapse with the postganglionic neurons in the following ganglia:
- paravertebral of the sympathetic chain located on both sides of the vertebral bodies;
- cervical;
- thoracic;
- rostral lumbar;
- caudal lumbar;
- pelvic; and
- the prevertebral ganglia, including the coeliac, aorticorenal, and superior and inferior mesenteric ganglia.
In addition, sympathetic preganglionic axons synapse with the chromaffin cells[7] of the adrenal medulla, which is functionally considered a ganglion of the sympathetic division of the ANS. The sympathetic division is involved in rapid reactions, like fight-or-flight, also known as the sympathoadrenal response of the body. The functions of the sympathetic division can be summarized as follows:
- increases heart rate and myocyte contractility facilitating blood flow to the skeletal muscles;
- causes vasodilation of the coronary vessels of heart;
- causes vasoconstriction of the gastro-intestinal track and skin diverting the blood flow to the skeletal muscles and the lungs;
- inhibits peristalsis;
- simulates breathing and relaxing the airways by dilating the bronchioles, which allows greater oxygen exchange;
- constricts the intestinal and urinary sphincters;
- in the eyes, dilates the pupil, allowing more light to enter;
- in coordination with the parasympathetic ANS, stimulates orgasm.
2.8 Summary
- The main functions of the nervous system are to sense the external environment and to exert control over the animal body, coordinating functions between different organs.
- It also connects with the endocrine system to regulate humoral signalling, maintaining the stability of the animal’s internal environment.
- The nervous system is a network of specialized cells that transmit chemical and electrical signals.
- Neurons – cells specialized for generating and transmitting electrical signals – are the functional units of the nervous system.
- The nervous system is also composed of non-neuronal cells, including glial cells. In vertebrate animals, nervous tissue of the brain and spinal cord contains capillary blood vessels in addition to neurons and glia.
- In vertebrate animals, the brain and spinal cord comprise the central nervous system (CNS).
- At early stages of embryo development, the CNS is composed by a simple neural plate that bends and folds to form a groove termed the neural tube.
- The anterior end of the neural tube develops into the brain and the posterior portion develops into the spinal cord. The neural tube bends and fold to form separate divisions. The anterior end of the neural tube develops into the brain and the posterior portion develops into the spinal cord.
- The neural tube forms cavities that are filled with the cerebrospinal fluid.
- The brain has three basic divisions: the hindbrain, midbrain, and forebrain.
- The hindbrain – subdivides into the myelencephalon and the metencephalon.
- The myelencephalon gives rise to the medulla oblongata.
- The metencephalon gives rise to the cerebellum and pons.
- The midbrain – does not subdivide and it is termed the mesencephalon.
- The forebrain – subdivides into the diencephalon and the telencephalon.
- The diencephalon gives rise to posterior forebrain structures, including the thalamus, hypothalamus, the posterior part of the pituitary gland and pineal gland.
- The telencephalon is the embryonic structure that gives origin to the cerebrum, which is divided into two symmetric hemispheres, the right and left hemispheres.
- The cerebrum consists of the basal nuclei, limbic system, and cerebral cortex.
- The cerebral cortex is the brain’s outer layer of neural tissue.
- The most recent development of the nervous system in mammals is the neocortex.
- In mammals, two visibly different regions of CNS can be distinguished: the grey matter, containing neuron cell bodies, and the white matter, containing tracts of myelinated axons.
- The spinal cord is a long, thin, tubular bundle of nervous tissue that transmits neural signals, through the medulla oblongata, between the brain and the rest of the body. The spinal cord is divided into five regions, cervical, thoracic, lumbar, sacral and coccygeal.
- The peripheral nervous system consists of all the nerves outside of the brain and spinal cord.
- The somatic nervous system is associated with the voluntary control of the skeletal muscles and consists of three parts: cranial, spinal and association nerves.
- The autonomic nervous system controls most of the involuntary visceral functions, those that are below the level of consciousness.
- The efferent nerves of the autonomic nervous system are further divided into two divisions, the sympathetic division and the parasympathetic division.
- Both divisions act on the same organs with opposing actions, but not always necessarily, because they also cooperate in regulating some functions.
- The functions of the ANS are integrated by the hypothalamus that receives regulatory signals from other parts of the brain.
2.9 Glossary
action potential – a self-regenerating wave of altered membrane potential that sweeps along a biological membrane of excitable cells such asneurons and muscle cells.
afferent – carrying towards or inward.
afferent nerve –
amygdala –
anaxonic neuron – a neuron that has no distinct axon; The dendrites perform some of the same functions of an axon.
ANS – autonomic nervous system.
arachnoid mater –
astrocyte –
autonomic nervous system –
axon –
axon hillock –
basal nucleus –
nuclei
bipolar neuron –
brain –
cell body –
central nervous system –
cerebellum –
cerebral cortex –
cerebral ganglia –
cerebrospinal fluid –
cilium –
circuit –
Cnidaria –
CNS – central nervous system
cranial nerve –
Ctenophora –
dendrite –
diencephalon –
dorsal root ganglion –
ganglia
dura mater –
efferent – carrying away or outward.
efferent nerve –
electrical capacitance –
electrical resistance –
ependymal cell –
ganglion –
ganglia –
glia – Any type of cell that isn’t a neuron and is found in the nervous system but nowhere else (see ‘glial cell’ below)
glial cell – Non-neuronal cell unique to the nervous system. Nervous systems typically contain several different kinds of glial cells.
grey matter –
hippocampus –
hypothalamus –
interneuron –
limbic system –
medulla oblongata –
membrane potential – the difference in electrical potential (voltage) between the two sides of a biological membrane.
meninges –
metencephalon –
microglia –
mixed nerve –
motor neuron –
multipolar neuron –
myelencephalon –
myelin –
myelin sheath –
neocortex –
nerve –
nerve tract –
neurohypophysis –
neuron –
ocellus –
ocelli
olfactory bulb –
oligodendrocyte –
pacemaker –
parasympathetic division –
peripheral nervous system –
pia mater –
pigmented cell –
pituitary gland –
PNS – peripheral nervous system
pons –
pseudounipolar neuron –
satellite cell –
Schwann cell –
sensory neuron –
SLUDDSA – Salivation, Lachrimation, Urination, Digestion, Defecation, and Sexual Arousal
soma –
somatic nervous system –
spinal cord –
spinal nerve –
sympathetic division –
synapse –
telencephalon –
thalamus –
unipolar neuron –
ventricle –
white matter –
- Neurosecretory cells are neurons that release chemical signals into the bloodstream instead of directly to another cell by way of a synapse. ↵
- Confusingly, the brain of vertebrate animals contains a class of bipolar neurons called ‘unipolar brush cells’. ↵
- Electrical resistance is the difficulty of an electrical charge to pass through a conductor. ↵
- Electrical capacitance is the ability of a body to store an electrical charge. ↵
- The synapse is a junction where axon terminals meet a neuron, muscle cell, or gland, and permit the passage of electric or chemical signals. ↵
- Arousal is the physiological and psychological state of being awake. It involves activation of a set of interconnected nuclei that are located throughout the brainstem, the autonomic nervous system and the endocrine system, leading to increased heart rate and blood pressure and a condition of sensory alertness, mobility and readiness to respond. ↵
- Chromaffin cells are cells characterized by their affinity for chromium salt used to stain the adrenal tissue. ↵