Indicators of Hepatic Dysfunction
Severe pathology can be present within the hepatobiliary system without impairment of hepatic function. When about 70% or more of the liver is non-functional, due to congenital or acquired hepatic disease, the liver is unable to carry out its metabolic, synthetic, and detoxification tasks. Important clues of hepatic dysfunction may be noted on a routine biochemical panel. These include: hypoglycemia, low urea, hypoproteinemia (particularly hypoalbuminemia), and hypocholesterolemia. Increased activities of enzymes that indicate hepatocellular leakage and cholestasis, and serum bilirubin alterations are more variable since hepatocellular injury and biliary stasis are not necessarily present with hepatic failure/dysfunction. Because cholestasis can be due to both hepatocellular (intrahepatic) pathology and biliary tract (extrahepatic) pathology, serum bilirubin is considered a liver function test as well as an indicator of cholestasis, and must be interpreted in relation to other findings. Specialized tests, such as bile acids and blood ammonia, can provide additional information about hepatic function.
Glucose
Although most animals with hepatic disease are normoglycemic, hypoglycemia may be seen, particularly with end-stage hepatic failure or portosystemic shunts. This is explained by impaired hepatic gluconeogenesis, glycogenolysis, or both. Post-sampling/in vitro glycolysis should be ruled out before pursuing other causes of low serum glucose, particularly if the patient is not displaying clinical signs normally expected with hypoglycemia. Fasting or anorexic animals are generally able to maintain their serum glucose concentrations within RI, so lack of food intake is not usually considered a differential for hypoglycemia. A possible exception would be toy breed puppies; however, the hypoglycemia seen in these animals relates not just to feeding patterns, but also to a lack of glycogen stores in the immature liver. Although less common than hypoglycemia accompanying hepatic failure, hyperglycemia may occur in some animals due to decreased glucose uptake by dysfunctional hepatocytes, resulting in prolonged postprandial hyperglycemia.
Urea
Urea is synthesized from ammonia that is delivered to hepatocytes from the intestines via the portal circulation. With hepatic dysfunction or shunting of portal blood to the systemic circulation, blood ammonia increases and urea synthesis by the liver decreases.
Protein
Most serum proteins, except immunoglobulins, are produced by the liver. When the functional capability of the liver is reduced by 60-80%, hypoalbuminemia, in particular, may develop. If serum albumin is reduced to about 10-12 g/L (1.0 – 1.2 g/dL), edema may develop. However, ascites may occur with hepatic disease in animals that do not have significantly reduced serum albumin concentrations. Portal and lymphatic hypertension related to hepatic pathology may explain the presence of ascites under these circumstances.α-and β-globulin synthesis is expected to be reduced along with albumin. γ-globulins may be within RI, but occasionally are increased. Presumably, impaired Kupffer cell antigen removal in the liver may lead to increased exposure of the immune system to foreign antigens and hence, increased γ-globulin synthesis. In these cases, serum protein electrophoresis may reveal fusion or bridging of β and γ-globulins. This pattern is due to augmented IgA and/or IgM synthesis, resulting in poor separation between β and γ fractions. Although β-γ bridging can be seen with certain types of hepatic disease, non-hepatic inflammation and infections have also been associated with this pattern.
Cholesterol
Serum cholesterol can be within the RI, high, or low with liver disease. Cholestatic liver disease is often associated with hypercholesterolemia due to decreased flow of bile, of which cholesterol is a major component. However, because the liver synthesizes cholesterol, significant hepatic dysfunction can result in hypocholesterolemia. Hypocholesterolemia is most commonly seen with end-stage liver failure and portosystemic shunts (PSSs).
Coagulation Proteins
Most of the coagulation proteins are synthesized in the liver. In addition, Factors II, VII, IX, and X require vitamin K for carboxylation which is necessary for calcium binding and hence, for their participation in coagulation reactions. Impaired bile flow can lead to decreased vitamin K assimilation and, therefore, reduced availability of functional vitamin K-dependent coagulation proteins. Also, reduced synthesis of coagulation proteins due to hepatic failure can lead to prolonged coagulation times, which may or may not be manifested as clinical bleeding. In addition, the liver is responsible for synthesizing many anticoagulant proteins and for clearance of activated coagulation factors. Therefore, thrombosis can also occur with liver failure. Animals with liver disease may experience complex hemostatic disorders involving both bleeding and thrombotic tendencies. Prothrombin time, partial thromboplastin time, fibrin degradation products and D-dimers are often measured in animals with liver disease, particularly if surgery is being planned.
Bile Acids
Bile acids are excreted into the biliary tract, stored in the gall bladder (except horses since this species lacks a gall bladder), and expelled into the intestinal tract to aid in food digestion (Fig. 8.2). Although bile acids are synthesized in the liver, decreased concentrations are not seen with hepatic dysfunction, largely due to very efficient resorption from the ileum into the portal circulation and redelivery to hepatocytes. There is only a slight postprandial increase in bile acids under normal circumstances. Elevations in bile acids are seen with PSSs because bile acids are not delivered to hepatocytes for reuptake. Hepatic dysfunction, from other causes, can result in an inability of hepatocytes to reabsorb bile acids. Also, with cholestatic hepatic disease, bile acids may be retained instead of being excreted into the biliary tract. For this reason, bile acid test results can be difficult to interpret when cholestasis and hyperbilirubinemia are present, since elevations do not necessarily indicate hepatic dysfunction under these circumstances. In dogs and cats, baseline and postprandial blood samples are collected to assess the recirculation of bile acids. Feeding stimulates evacuation of the gall bladder which increases delivery of bile acids to the portal circulation, thereby challenging the enterohepatic reuptake of bile acids to a greater extent than the fasted/baseline test. Frequently (about 20–30% of the time), the fasting bile acids concentration is higher than the postprandial concentration. A likely explanation for this finding is that the gall bladder had emptied prior to collection of the fasting serum sample. The “fasting” sample, in this case, would actually be more representative of a postprandial sample. Maltese dogs often have much higher serum bile acids, when measured by the common enzymatic assay, than other dog breeds. In this breed, the ammonia tolerance test would be a better means to evaluate hepatic function.
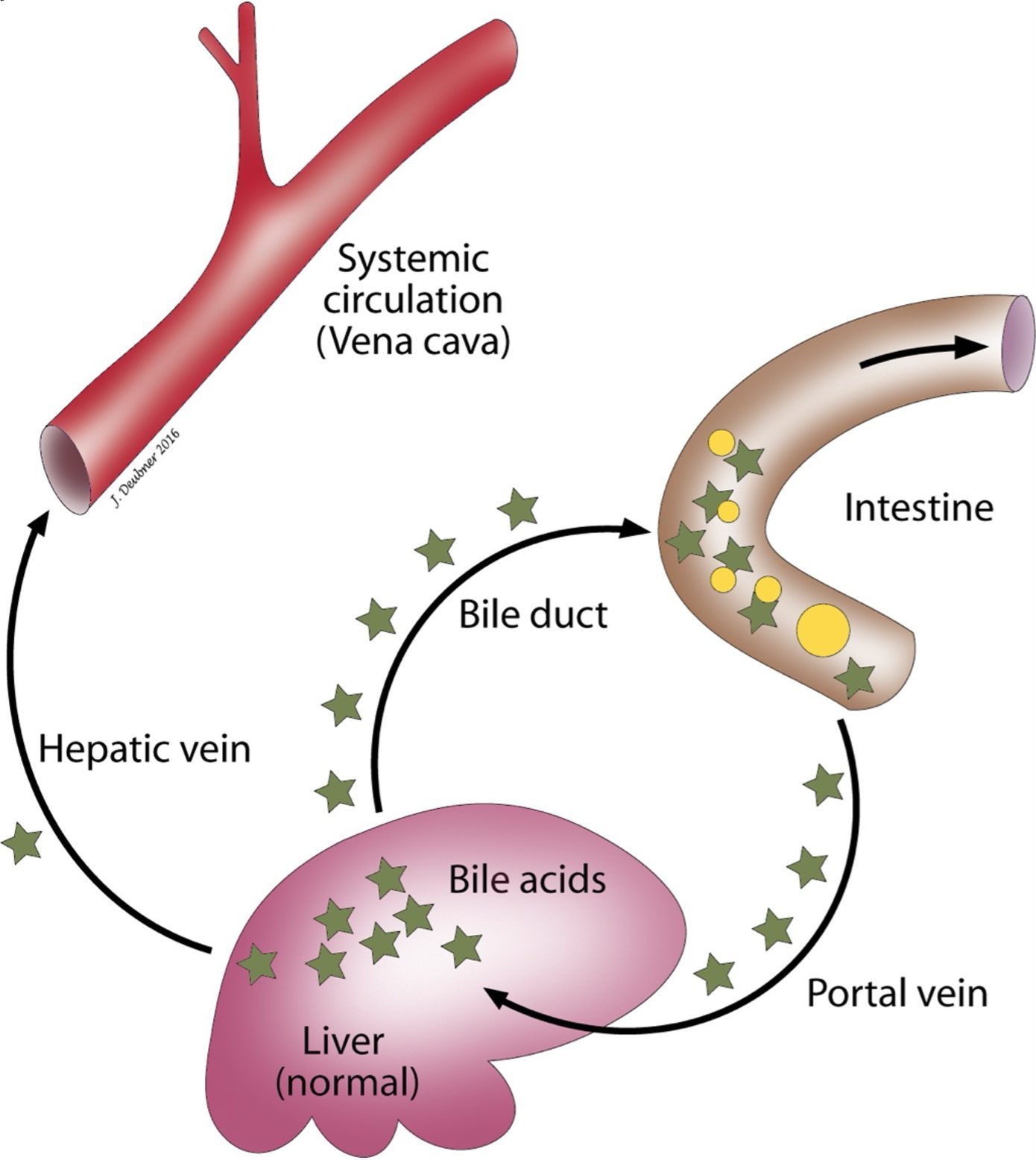
Ammonia
Deamination of amino acids is required so that they can be used as an energy source or converted into fats and carbohydrates (Fig. 8.3). Most of the deamination process occurs in the liver. Ammonia is a by-product of this deamination. Also, large amounts of ammonia are formed by bacteria in the intestine and absorbed into the portal circulation. In the liver, ammonia is removed from the blood and enters the urea cycle. Urea is subsequently eliminated from the body via the kidneys. With hepatic dysfunction, the ability of the liver to synthesize urea from ammonia is impaired and blood ammonia concentrations increase. Excessive ammonia in the circulation is highly toxic and is partially responsible for neurological signs seen in animals with hepatic diseases such as PSSs and end-stage hepatic failure. Animals with low serum urea concentrations due to hepatic disease would be expected to have elevated blood ammonia concentrations. Fasting blood ammonia may be significantly above the RI or blood ammonia may only be elevated when the animal is challenged with an excess load of oral ammonium chloride. There is a degree of risk in administering an ammonium chloride load to an animal with compromised hepatic function. Many laboratories recommend that if the patient is exhibiting neurological signs, the fasting ammonia concentration should be determined before proceeding with ammonium chloride administration. The challenge test is not indicated if the fasting blood ammonia concentration is above the RI. Sample collection procedures and technical aspects of plasma ammonia determination are more demanding than the more commonly used bile acids test. Blood ammonia measurement can be particularly useful in cases where cholestasis precludes interpretation of the bile acids results and, as mentioned earlier, in Maltese dogs. Irish wolfhound puppies nearly always have transient high plasma ammonia concentrations which normalize by adulthood. This finding is thought to relate to a urea cycle enzyme defect which self-corrects, with maturation, through development of alternate pathways. Bile acid tests are unaffected in these dogs.
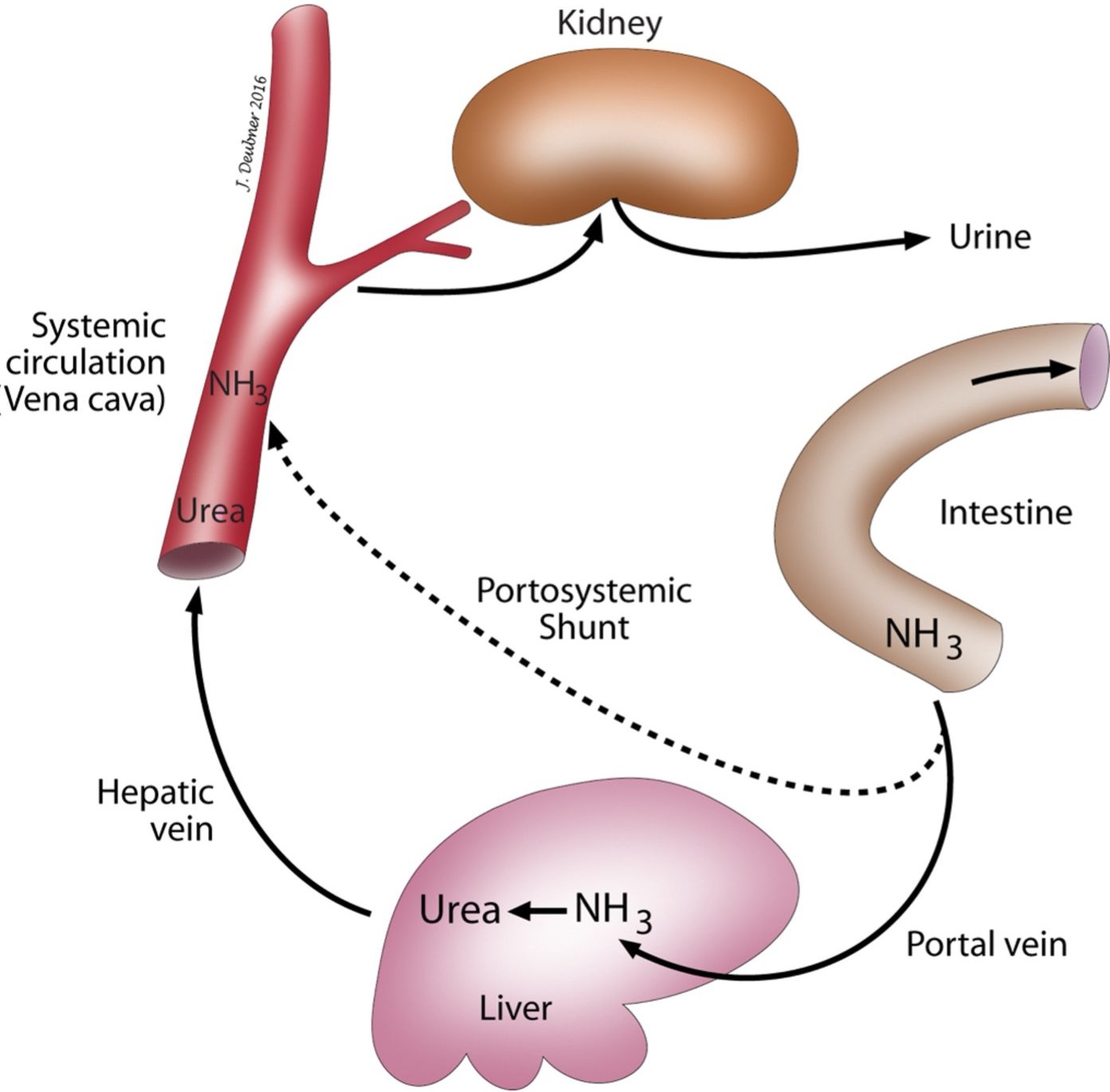
Formed in the liver from cholesterol and excreted into the intestine to aid the digestion of fats. Measurement after a meal can be useful in evaluating hepatic function.
Technique for separating and quantitating proteins in fluids, e.g. serum or urine.
Lipid used to form cell membranes, steroid hormones, bile acids, and vitamin D.
Any of several plasma components essential for secondary hemostasis.
The end product of coagulation, produced by the conversion of fibrinogen.
Fibrin fragments resulting from fibrinolysis.