Digging In
8 Soil Classification and Distribution
Daniel J. Pennock
LEARNING OUTCOMES
On completion of this chapter, students will be able to:
- Explain the role that diagnostic horizons play in classification of soils under the Canadian System of Soil Classification (CSSC)
- List the seven soil-forming factors that control the distribution of soils at national and local levels
- Explain how the CSSC uses soil pH to distinguish between acidic parent materials derived from igneous rocks and neutral-alkaline parent materials derived from sedimentary rocks
- Identify the three main soil orders (Brunisolic, Podzolic, and Luvisolic) typically associated with forested landscapes in Canada
- Understand the effect of permafrost layers on Arctic and tundra soils of the Cryosolic order
- Identify the three main soil orders (Chernozemic, Solonetzic, Vertisolic) typically associated with grassland landscapes
- Explain how the two orders of wetland soils (Gleysolic, Organic) differ
- Understand how the usefulness of a given soil survey for land use planning is determined by the scale of the survey
- Identify the major classes used in the Canada Land Inventory
INTRODUCTION
Classification and mapping of soils in Canada was a major activity in soil science throughout the latter half of the 20th century and the maps that resulted from this program are still widely used in many sectors. As well, mapping of soils at intensive scales for activities such as pipeline development, mine reclamation, and soil contamination remediation are major activities by private sector consultants in Canada. Hence, an understanding of the classification system used in Canada and how soil maps can be used is essential for many soil scientists.
The placement of soils into the correct class is based on the horizons that we can see in the soil profile. Distinctive sets of soil horizons result from the response of the soil-forming processes (discussed in Chapter 2) to soil forming factors. These sets of soil horizons differ from point to point over short distances – within a field, for example – and even more so over large distances such as a county, rural municipality, or province. The sets of horizons are the basis for classifying individual soils into the taxonomic system used in Canada, the Canadian System of Soil Classification (CSSC) (Soil Classification Working Group, 1998). Moreover, the distribution of soil horizons and soil properties is not random – they are responding to a set of environmental controls and once we understand the controls we can predict the distribution of soils and hence map their distribution.
Soil maps are a key source of information in many types of land use planning. The usefulness of a map for any particular purpose depends on the scale of the map – the more detailed the scale, the more certain we can be that map accurately reflects the actual distribution of soils at the site. Evaluating the reliability of soil maps (or surveys) requires a good understanding of the interplay between map scale and map accuracy. Digital Soil Mapping, which draws upon sophisticated computer and remote sensing tools, is increasingly being used to produce soil maps at all scales. Digital map products allow the accuracy of the map to be reliably estimated (see Chapter 17 for more information on Digital Soil Mapping).
SOIL HORIZONS AND THE PEDON
An initial stage in the evolution of any natural science is the naming (or classification) of the objects under study. Often there is an informal name that will differ regionally or in different language groups (e.g., the Blue Jay or Jack Pine) and a formal, scientific name that is used internationally (at the species level, Cyanocitta cristata and Pinus banksiana). The classification system used to identify and name the objects is formally called a taxonomic system.
Soils are unlike birds or trees insofar as there are no naturally occurring soil individuals (such as an individual tree or bird). The soil forms a layer on the Earth’s surface whose properties vary continuously through space – the soil at any given location will be different (although often in very subtle ways) from the soil even a footstep away. Moreover, only the uppermost millimeter of the soil is even visible to us – the vast majority of the soil is underneath the ground surfaces where it is invisible to even sophisticated remote sensing devices.
Given this continuous variation in soil properties, the definition of a basic soil individual is therefore arbitrary. For the purposes of classification soil scientists developed the concept of a pedon – formally defined in Canada as:
“It [the pedon] is the smallest, three-dimensional unit at the surface of the earth that is considered as a soil. Its lateral dimensions are 1 m if ordered variation in genetic horizons can be sampled within that distance or if these horizons are few and faintly expressed.” (Soil Classification Working group, 1998; p.9)
Typically, the way we observe the soil is to dig a hole in the ground, which is referred to as a soil pit. The pit is the means for describing the soil profile and for taking samples from the soil which we analyze in the laboratory.
Mineral and Organic Soil Horizons
As we learned in Chapter 2, soils are composed of one or more distinct layers, which are formally called soil horizons. These layers may differ from each other in terms of their colour, structure, texture (the particles which compose it) or many other properties. In every soil classification system, these layers are assigned distinctive alphabetic symbols as a form of shorthand for their characteristics.
The horizon description system begins by splitting soil horizons into two distinct groups: mineral and organic horizons. Organic horizons are those which contain 17% or more organic carbon by weight; mineral horizons have less than 17% organic carbon by weight.
The three master mineral horizons are the A, B, and C horizons.
A horizon: The A horizon occurs at or near the soil surface. In the CSSC two very distinct bundles of processes can form A horizons. The first is the addition, mixing, and transformation of organic matter (OM) to form an organically enriched mineral horizon (Ah). The second bundle involves the bleaching of the horizon by acids and (in many cases) the loss of mobile soil components such as clay, OM, iron, and aluminum. The loss of soil material is called eluviation, and these are classified as Ae horizons. The CSSC is unusual for having two very different types of A horizons – in the major international soil classification systems, the eluvial horizon is assigned an uppercase E, not an Ae, label as is used in the CSSC.
B horizon: The B horizon is a layer that has undergone substantial change from the parent material. In aerobic soils this may be due to the formation of red surface coatings on mineral grains, deposition of materials (clay, OM, iron, aluminum) from the Ae, loss of highly weatherable carbonate or salts, or the formation of distinct soil structural units. In anaerobic soils, water saturation can lead to processes that form the gley soil properties, such as dull coloration or the presence of mottles.
C horizon: In many cases (especially in parent materials high in sand) the C horizon has experienced little or no soil formation and closely resembles the parent material the soil formed in (assuming that no layering of parent materials occurs in the profile). In soils formed in carbonate or salt-rich parent materials, dissolved ions from these minerals that originate from the A and B horizons may re-form new, secondary minerals in the C horizon.
The main organic soil horizons are the O horizons and the LFH horizons. The O horizons are organic layers formed in bogs, fens, swamps, and marshes and are informally called peat layers. They are further subdivided based on their degree of decomposition into highly fibrous layers of recognizable plant origin (Of for fibric), layers of intermediate decomposition (Om for mesic), and highly decomposed material (Oh for humic).
The LFH horizons occur from the accumulation of leaves, twigs and woody materials on the mineral soil surface in forests or shrublands and which overlies a mineral soil. The L layer is from readily recognizable leaf litter, the F from moderately decomposed organic material (known as folic material), and the H from highly altered humic material.
THE CANADIAN SYSTEM OF SOIL CLASSIFICATION
Taxonomy is the science of classification, and the CSSC is a rigorous taxonomic system. The System is a comprehensive method for assigning pedons or profiles to the soil classes; if the system is correctly used, a pedon can only be assigned to one specific class. The System is also a hierarchical system: each group occupies a distinct position within the overall system. The major levels used in the classification are shown in Table 8.1. The first three levels (order, great group and subgroup) are used in soil classification in the field whereas the final three (family, series, and phase) are primarily used in soil mapping.
Table 8.1. Taxonomic levels in the Canadian System of Soil Classification ordered from highest (the most general) to lowest (the most specific)
Class | Description |
---|---|
Order | Orders are based on properties of the profile that reflect the nature of the overall soil environment and the effects of the dominant soil-forming process. |
Great Group | Great groups are subdivisions of each order. The great groups reflect differences in the strengths of the major processes or a major contribution of a process in addition to the dominant one. |
Subgroup | Subgroups are subdivisions of great groups. Profiles are differentiated on (1) how closely they correspond to the central concept of the great group (termed Orthic); (2) transitional stages grading towards soils of another order; and (3) additional special features within the profile. |
Family | Families are used to group series (see below) of the same subgroup that have similar response to management, engineering and related uses. The family level is seldom used in Canada. |
The assignment of a given soil profile to its taxonomic class is done by comparing the properties of the horizons in the field to a list of distinctive characteristics, called diagnostic properties. These diagnostic properties are the basis for classification of any pedon into the formal classes of the CSSC (Table 8.2).
Table 8.2. Diagnostic horizons and main characteristics of the ten orders in the Canadian System of Soil Classification
Order | Diagnostic | Nature of the soil environment and dominant soil-forming process |
---|---|---|
Horizon | ||
Chernozemic | Ah (humus), | Grassland soil whose diagnostic horizon is formed by high levels of organic matter additions from the roots of grasses and turbation by burrowing animals. |
Ap (ploughing) | ||
Ahe (humus + eluviation) | ||
Solonetzic | Bn (sodium) or Bnt (sodium + clay) | Grassland soil with high sodium levels in the B horizon; usually associated with a clay-rich B horizon and often with saline C horizon material. |
Podzolic | Bf (iron) or | Forest soil normally associated with coniferous vegetation on igneous-rock derived parent materials. High acidity in the A horizon results in formation of a bleached Ae horizon and formation of organic-mineral complexes that are transferred to the B horizon. |
Bh (humus) | ||
Luvisolic | Bt (clay) | Forest soil found in areas with parent materials derived from sedimentary rocks. Dominant property is higher clay content in the B than the Ae horizon caused (in part) by loss of clay from the Ae horizon and its deposition in the Bt horizon. |
Brunisolic | Bm (minimal transformation) | Forest soil whose properties are not strongly enough developed to meet the criteria for the Luvisolic or Podzolic Orders. |
Gleysolic | Bg, Cg (gleying) | Wetland soil found throughout Canada wherever temporary or permanent water saturation cause formation of gleyed features (mottles, blue-grey colours) in the profile. |
Regosolic | No B horizon or B horizon less than 5 cm thick | A minimally developed soil found throughout Canada wherever conditions prevent the formation of B horizons (unstable slopes, sand dunes, floodplains etc.). |
Vertisolic | Bss or Css | Grassland soil associated with high clay glacio-lacustrine landscapes; characterized by shrinking and swelling of clays. |
(slickensides) | ||
and Bv (vertic mixing) | ||
Cryosolic | By, Cy, (cryoturbation) | Soil of arctic and tundra regions with permanently frozen layers in the soil profile. |
Cz (permafrost) | ||
Organic | O (Organic) | Wetland soils associated with the accumulation of organic materials (peat) in water-saturated conditions.They are most commonly associated with the Boreal forest and tundra regions. |
ENVIRONMENTAL CONTROLS ON SOIL DISTRIBUTION
In the middle of the 20th century several pedologists independently developed functional relationships between the properties of soils and the environmental controls (or factors) on the processes that form the properties. The best known of these relationships is by the American soil scientist Hans Jenny in his famous book the Factors of Soil Formation (1941). At about the same time, the great Canadian pedologist Joe Ellis identified seven factors in his book the Soils of Manitoba (1939). The work of the two authors overlapped for several of the factors but each statement had unique elements and combining them gives us a comprehensive set of soil-forming factors:
- Climate: the temperature and moisture within the soil.
- Organisms: the vegetation, animals, and microbes that affect the soil.
- Parent material: the mineral matter in which the soil is formed, and which in turn affect the texture, water retention capacity, and the mineral reserve.
- Topography (or relief): the shape of the land surface.
- Time: the duration of soil formation.
- Groundwater: the presence or absence of groundwater within the soil profile.
- Human activity: the soil-modifying effects of human use of the soil.
Note that groundwater and human activity are the two soil-forming factors that make a difference between Ellis’ and Jenny’s groupings of these factors. In Chapter 2 we saw how five of these factors (climate, organisms, parent material, time, and human activity) have controlled the main bundles of soil-forming processes responsible for Canadian soils at the national level. The result of these bundles of soil-forming factors is to create a geographic zonation of soil orders in the different regions of Canada (Figure 8.1).

Local controls on soil distribution: the soil catena
At the more local level – for example in an individual hillslope or hillslope catchment – topography and groundwater have a major influence on the distribution of individual soil classes. As we learned in Chapter 2 much of the local topography in Canada is the result of glacial or post-glacial geomorphic processes and the basic shape of landforms does not change on human-time scales. The shape of the land surface has a very strong influence on the pattern of water movement both on the surface of the soil and laterally within the soil and this water movement and concentration is the dominant control on the local-scale pattern of soil formation (Figure 8.2).
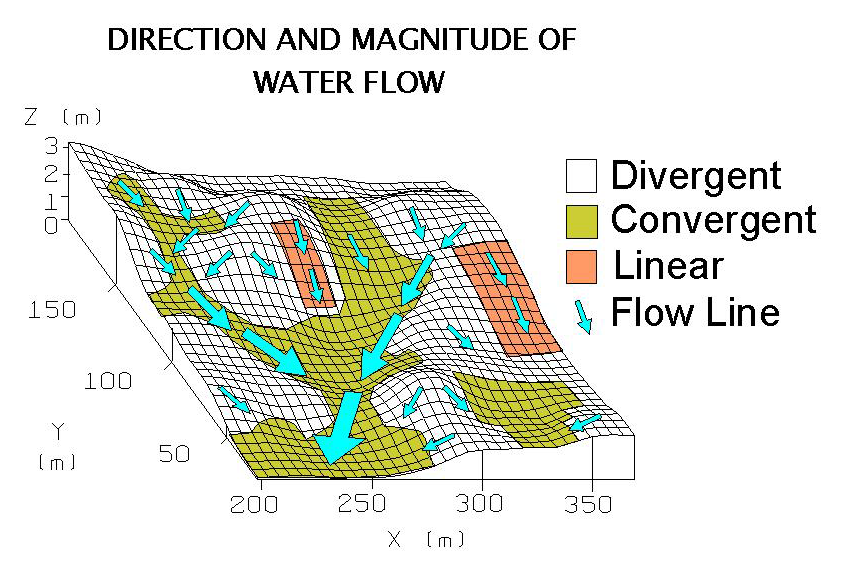
For dominantly aerobic soils (i.e., those with air-filled pore space) the greater the amount of water present in the soil profile, the greater the action of the soil-forming processes discussed in Chapter 2. For example, hydrolysis (literally the splitting of water molecule) releases the hydrogen ions that drive much of chemical weathering; hence, the greater the water available in the profile, the greater the chemical weathering that occurs. Water also transports mobile ions and dissolved OM out of the profile through the process of leaching; again, the greater the water flowing through the soil, the greater the leaching of solutes from the soil. Finally, microbial decomposition of OM (and the formation of humus) is greatest where optimum soil moisture and soil temperature conditions occur; drier soil moisture levels reduce the activity of the microbial community.
If, however, the pore space in soil profile is water-filled for extended periods of time, the soil becomes dominantly anaerobic and the chemical regime becomes dominated by redox processes (Chapter 5). Under those conditions, soil formation follows the gleyed pathway – reduction and mobilization of iron, formation of blue-grey soil colours, and slower (or no) decomposition of OM added to the soil.
Water movement in hillslopes can cause a major range in soil moisture conditions (and hence different soil classes) to occur over short distances (i.e., 10 m to a few hundred meters) (Figure 8.3). Generally upper slope positions shed moisture, and soil development will be minimally expressed in these positions. As soil moisture increases downslope, the degree of soil formation (expressed through soil thickness and development of soil horizons) will increase. Finally, a threshold may be passed where the soil becomes dominantly anaerobic and the gleying processes become dominant. This pattern of soil development along hillslopes repeats itself over many landforms, and the pattern is called a soil catena (catena is Latin for chain) – literally a chain of soils along the slope, genetically linked to one another.

As well as water transport within the soil there are also flows of water through sediments and even bedrock deep in the Earth’s surface. These groundwater flow systems can transport water (and solutes) over distances of hundreds of meters to many kilometers. In some topographical settings the pressures within the groundwater system cause the groundwater to move vertically upwards (or discharge) towards the soil surface. The groundwater can then be lost to the atmosphere due to evapotranspiration, and often any solutes in the groundwater precipitate out to form new mineral salts and carbonates. This is a major source of salinity in Canadian Prairiers. In areas of Canada with well developed river drainage systems, the groundwater more typically discharges into the stream system and soils affected by discharge are less common.
FOREST SOILS
Luvisols, Podzols, and Brunisols
The key taxonomic distinction in forested areas is between soils formed in generally coarse-textured glacial parent materials derived from acidic, base-poor (e.g., lacking magnesium, calcium, potassium and sodium) igneous rocks and those formed on glacial and post-glacial parent materials derived from high base-status sedimentary rocks. In the CSSC, the criterion used to distinguish between soils of the two types of parent materials is the soil pH (as measured using 0.01 M calcium chloride; see Chapter 5 for more information about soil pH and acidity). At the national level, soils developed on the igneous-rock derived parent materials have an acid pH < 5.5; soils on sedimentary rock parent materials have pH values in the neutral-alkaline range (i.e., ≥ 5.5). For the purposes of this chapter the soils in each group will be referred to by their pH range (i.e., acid vs. neutral-alkaline) rather than the full parent material description.
At the onset of soil formation four soil-forming processes begin to modify the upper portions of both types of parent materials: additions of OM to the soil surface, transformations of added by OM by the microbial community, chemical weathering of minerals in the parent material, and development of soil structure. In the earliest phase of soil formation, the alteration of the parent material is limited to a thin layer at the surface of the soil. In the CSSC, these soils are classified into the Regosolic Order (VandenBygaart, 2011). In most forested regions Regosolic soils only occur in unstable landscapes such as sand dune areas or active floodplains and are not readily mapped on a national-level map (Figure 8.4).

In acid parent materials, chemical weathering in the surface horizon begins to form a bleached horizon (Ae), which is underlain by a horizon with red coatings on the sand grains (Bm). Soils with acid Bm horizons over 5 cm thick are classified into the Brunisolic Order (Smith et al., 2011) (Figure 8.5). In some regions organic-iron or organic-aluminum complexes form in the upper soil and are deposited in the B horizon (i.e., podzolization). These horizons may be high in iron (Bf), aluminum, or organic materials (Bh) or all three materials (Bfh or Bhf), and are diagnostic of the Podzolic Order (Sanborn et al., 2011) (Figure 8.6).


The final major geographic distinction within the acid Brunisolic and Podzolic orders depends on whether earthworms or other burrowing animals are active in the surface horizon of the soils. Where earthworms are present the LFH horizons are mixed with the surface mineral layer and an Ah horizon occurs; where they are absent a sharp contact between the LFH and Ae horizon occurs. These differences are recognized in the great group level of the CSSC – acid Brunisols and the Podzols that have an Ah horizon at least 10 cm thick present are placed in the Sombric great group (Figure 8.7).
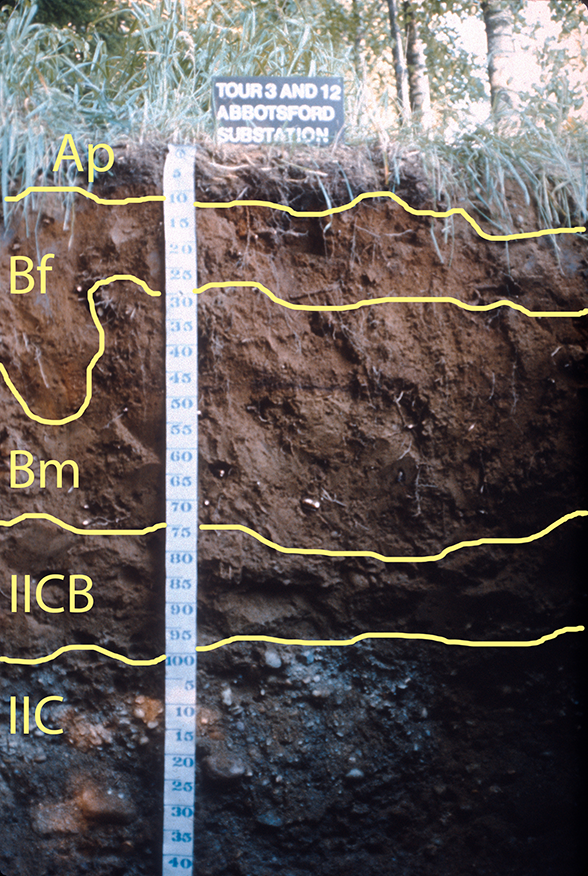
The regional climate is the major control on the distribution of acid Brunisolic and Podzolic soils (Figure 8.1). Generally Podzolic soils occur in wetter and warmer forested regions with acidic, igneous-rock derived parent materials, while Brunisolic soils can be found in drier and cooler regions of the continental interior (Smith et al. 2011, Sanborn et al. 2011). Podzolic soils are dominant in the Atlantic Maritime and the eastern portion of the Boreal Shield ecozone as well as throughout higher elevation portions of the Montane Cordillera and southern areas of the Boreal Cordillera. The base-poor, acidic classes of the Brunisolic order occur throughout the Taiga Shield ecozone and the Boreal Shield west from NW Ontario through the northern Prairie Provinces. They also dominate the Boreal Cordillera ecozone.
There are also large areas of the Boreal and Taiga Shield Ecozones where the dominant surface material (in terms of area covered) is rock (mapped as Unclassified in Figure 8.1). These are the iconic landscapes painted by Canadian artists such as Tom Thompson and the members of the Group of Seven in the first half of the 20th Century. There are pockets of sand and gravel (with Brunisolic or Podzolic soils) and Organic soils in depressions scattered throughout these landscapes, but the igneous or metamorphic rocks are the dominant type of surface material.
The genetic pathways for soils developed on neutral-alkaline parent materials derived from sedimentary rocks are very distinct from those discussed above but (somewhat confusingly) some of these soils are also classified into the Brunisolic Order. The first stage in the formation of these sedimentary-rock derived soils would involve the same four soil-forming processes (OM additions and transformations, chemical weathering, and structure formation). Because these parent materials typically have carbonates and more soluble salts present, the effect of weathering is to destroy carbonates and salts in the upper soil and transfer the ions released deeper into the soil (in the case of carbonates) or leach them completely from the soil (for the more highly soluble salts). This creates a carbonate- and salt-depleted B horizon, which is also designated a Bm horizon. There may also be reddening of the Bm horizon after removal of carbonates. Soils with a neutral-alkaline Bm horizon greater than 5 cm thick are again classified into the Brunisolic Order (Figure 8.8) but into different great groups (the Eutric and Melanic Great Groups) than the more acidic versions (the Dystric and Sombric Great Groups). More developed versions of these Brunisolic soils also begin to have bleached Ae horizons present.

The removal of carbonates allows clay particles in the upper soil to be detached from the soil mass, moved vertically in the soil, and deposited in the B horizon in the process of lessivage. This creates a textural contrast between the bleached Ae and the B; and the layer with higher clay content is denoted with a Bt horizon label. As discussed in Chapter 2, clay-enrichment from lessivage is very difficult to distinguish from textural differences caused by layering in parent materials and in practise all B horizons with higher clay contents than the Ae are given the Bt label. Soils with a Bt horizon are classified into the Luvisolic Order (Lavkulich and Arocena, 2011) (Figure 8.9).

As with the soils developed on acid parent materials, a further distinction is made between neutral-alkaline Brunisolic and Luvisolic soils with an organically enriched surface mineral horizon (Ah) caused by mixing (usually by soil-dwelling organisms) and those with a sharp contact between the Ae and LFH horizons. Again these differences are recognized in the CSSC at the great group level (Figure 8.10). The Gray Brown Luvisol and Melanic Brunisols have an Ah horizon greater than 10 cm thick present and the Gray Luvisols and Eutric Brunisols do not.
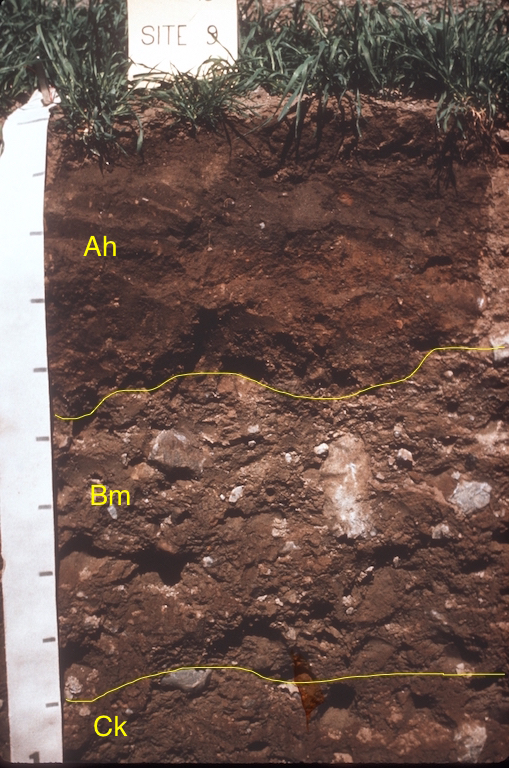
The greatest extent of Gray Luvisolic soils is in the Boreal Plains ecozone of the Prairie Provinces and southern portion of the Taiga Plains ecozone (Figure 8.1). This ecozone is dominated by mixed deciduous and coniferous forests. A similar mix of vegetation and soils occurs in the interior of British Columbia in the Montane Cordillera ecozone. The Gray Brown Luvisolic and Melanic Brunisolic soils are primarily found in the Mixedwood Plains ecozone in southern Ontario and in parts of the Atlantic Maritime ecozone in New Brunswick and Nova Scotia. A final area of Gray Luvisolic soils occurs on the areas of clayey glacio-lacustrine deposits in north-central Manitoba, eastern Ontario and western Quebec in the Boreal Shield ecozone.
WETLAND SOILS
Gleysols and Organic Soils
The two orders for wetland soils in the CSSC are differentiated based on the presence or absence of O horizons. Where the O horizon is at least 40 cm thick, wetland soils are classified into the Organic order; where the O horizon is less than 40 cm or absent altogether, wetland soils are classified into the Gleysolic order (Figure 8.11). As we saw in Chapter 2, the distribution of wetland soils is controlled by the duration of water-saturated or anaerobic conditions and the effect of anaerobic conditions on the decomposition of organic materials and soil chemical environment.

Soils of both orders occur in every province of Canada but they rarely are dominant in the landscape (Figure 8.1). More commonly they are found in depressional positions in a landscape where water accumulates and saturated soil conditions occur. Hence, these soils are common in soil catenas throughout Canada but are rarely the dominant soil order present.
Soils of the Organic order dominate the Hudson Plains ecozone, which is one of the largest wetland areas in the world (Kroetsch et al., 2011). They also occur in large areas in the Boreal Plains ecozone at the contact with the Boreal Shield ecozone (Chapter 2; Figure 2.11). They also occur throughout the forested landscapes of Canada in depressional sites or in areas of sluggishly moving water flow.
Soils with organic layers greater than 40 cm occur in two other settings in Canada. The first is in the wet, temperate rainforests of coastal British Columbia, where the very high precipitation levels (typically greater than 3000 mm per year) cause water saturation and retards the decomposition of leaf litter and other aboveground forest biomass (Fox and Tarnocai, 2011). This leads to the buildup of this material (generally termed folic material) and soils in this region are classified into the Folic great group of the Organic Order (Figure 8.12). There are also substantial areas of soils with permafrost in the southern Yukon and south-west NWT that have an organic layer greater than 40 cm thick. These soils are discussed in the following section on Arctic and Tundra soils.
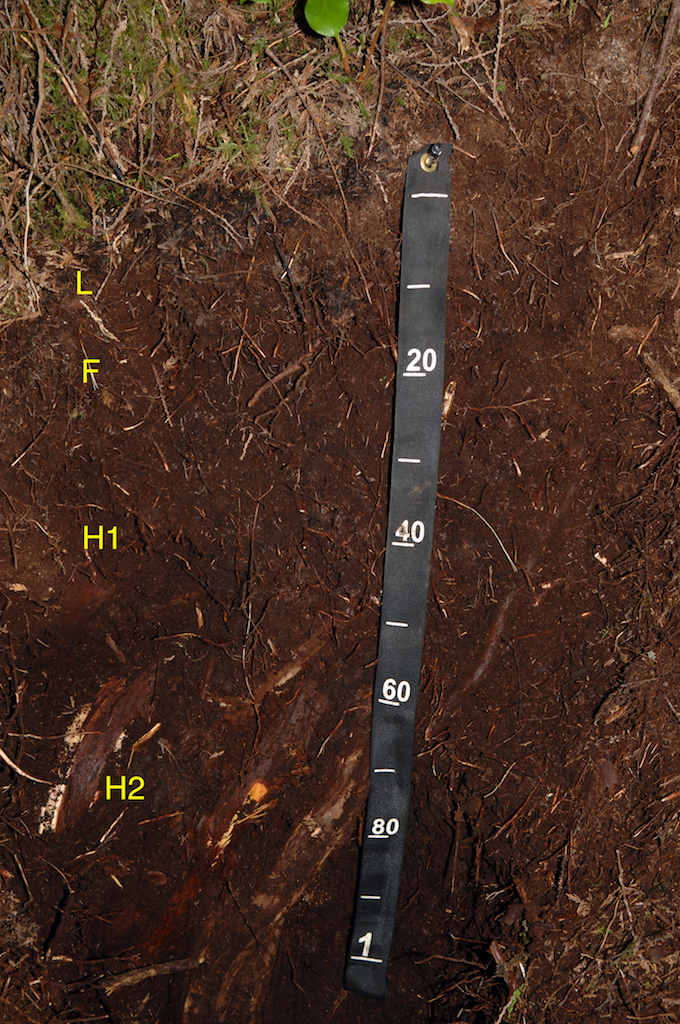
Gleysolic soils are mineral soils that exhibit evidence of gleying conditions: blue-grey colours, reddish mottles and low soil chromas indicative of prolonged water saturation (Bedard-Haughn, 2011) (Figure 8.13). Horizons with these features are assigned a lowercase g label. This label can be assigned to A horizons (Aeg) or to a range of B and C horizons. The Ah horizon is typically too dark to allow visible expression of the gley properties.

Soils of the Gleysolic order are only dominant in small areas of Canada such as in parts of the Mixedwood Plains ecozone in southernmost Ontario and south-west Quebec (Figure 8.1). In both of these areas, the Gleysolic soils occur on high clay glacio-lacustrine or glacio-marine parent materials through which the precipitation drains very slowly, creating anaerobic conditions and Gleysolic soils (Bedard-Haughn, 2011). An example of the catenary position of Gleysolic soils is shown for the Huron catena in Southern Ontario (Figure 8.14). The lower slopes in this landscape have silty clay loam and clay loam parent materials, while the upper slopes have loams or sandy loams. Water concentrates in the lower slopes and water movement (or drainage) through the finely textured soil is very slow. This leads to prolonged saturation and the creation of gley properties. The dominant soil (in terms of area) is Luvisolic, hence the area would appear on the national map as Luvisolic. Digital soil mapping (as discussed in Chapter 14) allows for the creation of digital maps where the individual soils can be more readily identified and mapped.
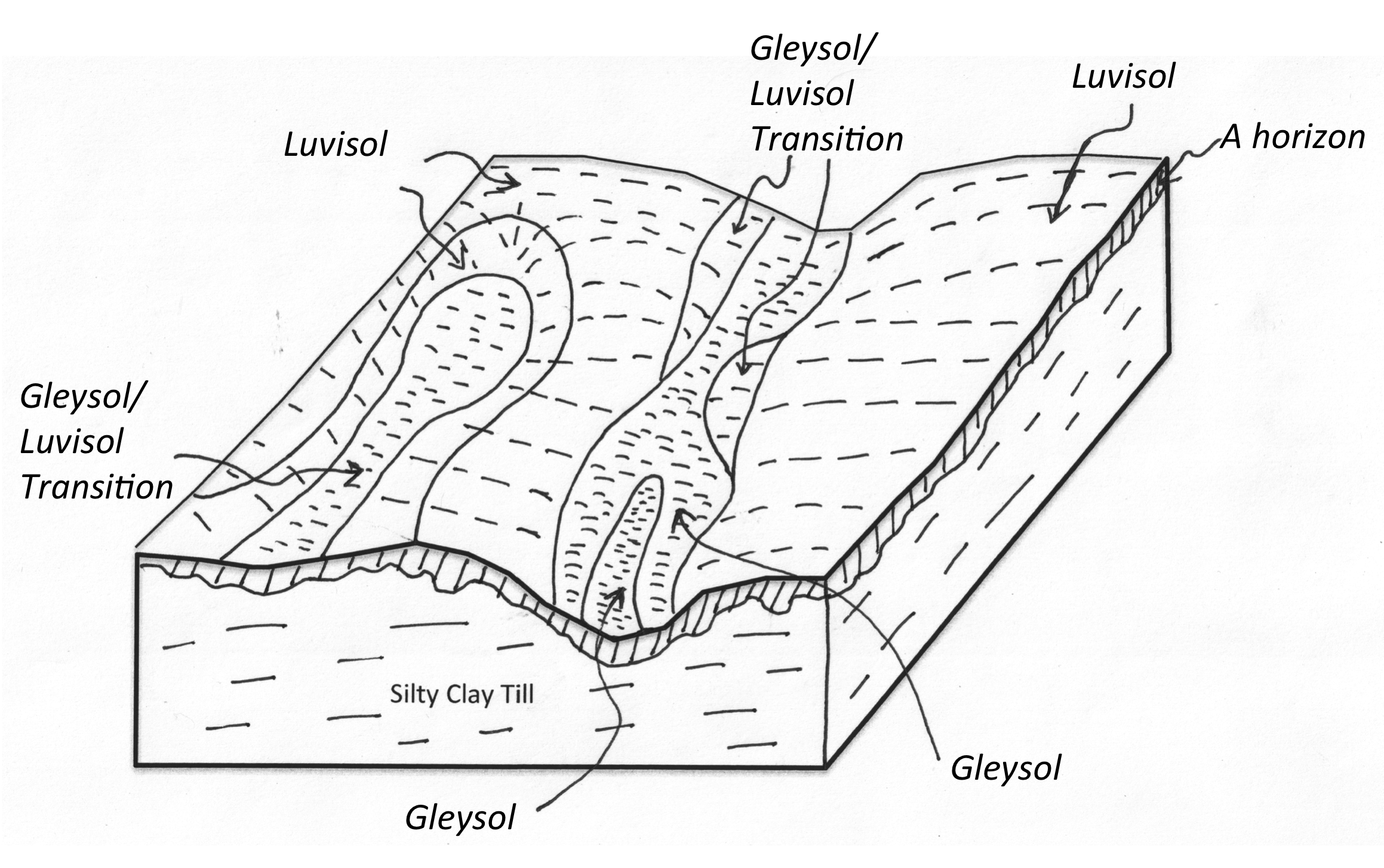
ARCTIC AND TUNDRA SOILS
Cryosols
In the CSSC soils with a permafrost layer within 1 to 2 m of the soil surface are classified into the Cryosolic order. Cryosolic soils are the dominant soil in Canada, covering about 2.5 million km2 or about 35% of the soil area of Canada (Tarnocai and Bockheim, 2011) (Figure 8.1). Cryosolic soils completely dominate the Arctic Cordillera and Northern Arctic ecozones and the northern portions of the Taiga Cordillera, Plains and Shield ecozones. In the southern portions of the Taiga ecozones, the permafrost becomes discontinuous and the Cryosolic soils intermingle in the landscape with soils of the Brunisolic order.
The Cryosolic soils follow three broad pathways, which are recognized at the great group level in the CSSC. In the first pathway, the growth of ice lenses in the soil causes considerable mixing of soil material, which disrupts horizons and creates profiles with a complex pattern of discontinuous horizons. These soils are classified into the Turbic great group of the Cryosolic order, which is the dominant class in terms of area (Figure 8.15). Describing horizons in the Turbic Cryosols can be complex due to the discontinuous nature of the horizons (Figure 8.16). The diagnostic suffixes for the Cryosolic order are the y suffix (for horizons affected by cryoturbation as shown by disrupted and broken horizons) and the z suffix (for a frozen layer). Both of these suffixes can be used with any of the master horizons (i.e., A, B, C, or O). Pockets of Ah horizon and Bm horizons also often occur in Cryosolic landscapes.
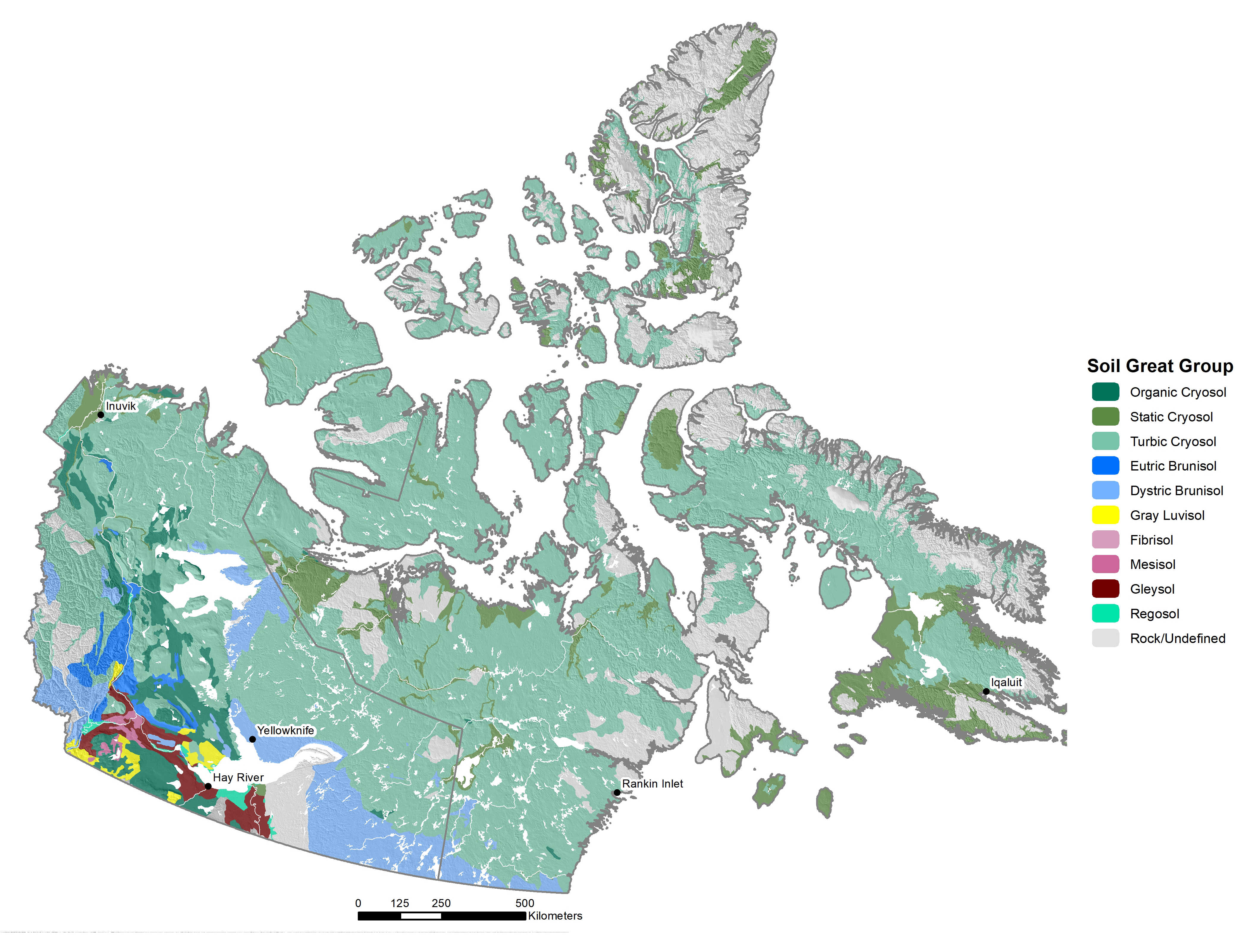
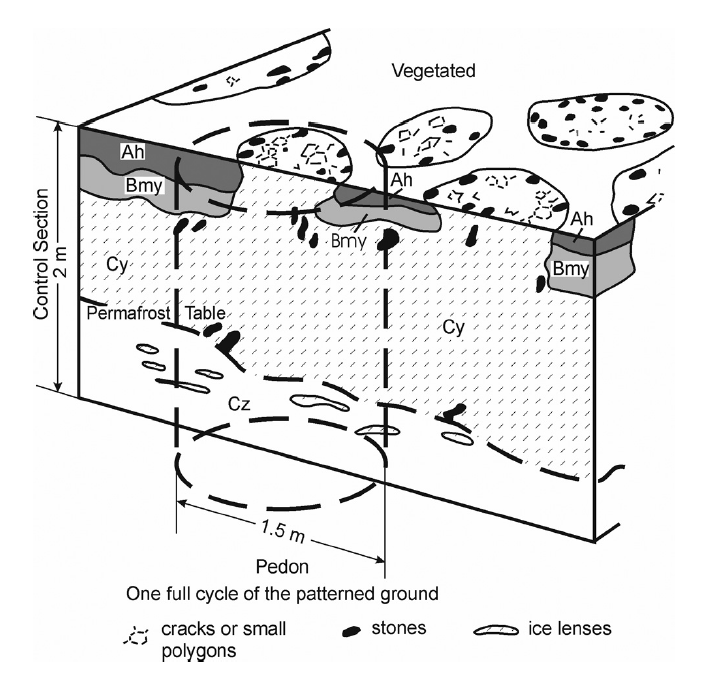
In some smaller areas along the coastlines of the regions where the soil parent materials are too coarse to permit water transfer to form ice lenses, mixing due to ice action does not occur. These soils are classified into the Static great group (Figure 8.17). Finally, in the (relatively!) more temperate areas of the southern Yukon and south-west NWT, we can find Cryosols with an organic layer thicker than 40 cm. These are classified into the Organic great group of the Cryosolic order (Figure 8.15).

GRASSLAND SOILS
Chernozems, Solonetz, and Vertisols
Three soil orders of the CSSC are found only in grassland or forest-grassland transition regions: the Chernozemic, Solonetzic, and Vertisolic orders (Anderson and Cerkowniak, 2010). The Chernozemic order is the dominant order, and the Solonetzic and Vertisolic orders are primarily associated with particular parent materials (Figure 8.1). Overall, the grassland regions are dominated by loam and clay loam till parent materials: 35.1 million hectares (Mha) of loam to clay loam till out of a total of 53.1 Mha of farmland in the region (Pennock et al., 2011). All areas of the grasslands have a significant soil moisture deficit ranging from 200 mm in the Brown soil zone in southern Saskatchewan to 80 mm in the transition grassland-Mixedwood forest zone of the Boreal Plains ecozone (Pennock et al., 2011).
Grassland soils in Canada are overwhelmingly found only on neutral-alkaline parent materials. They are characterized by high base saturation and the presence of carbonates and more soluble salts in the parent materials. In the first stage of soil formation the growth of grasses (with their lush root mass) and mixing by soil-dwelling mammals transforms the surface mineral soil into an Ah horizon, with the suffix h indicating humus formation. The combined action of hydrolysis and the organic acids produced in this layer begin to dissolve the salts and carbonates below the Ah horizon and a leached Bm horizon forms along with the start of a layer of re-formed calcium carbonate (Cca) deeper in the soil. When the Ah and Bm are very thin these soils would be classified into the Regosolic order (Figure 8.18), but within a few hundred years of soil formation they would become classified into the Chernozemic order.

The diagnostic horizon of the Chernozemic order is an Ah horizon that has dark soil colour (due to OM enrichment), high levels of bases (especially calcium), and is at least 10 cm thick (Figure 8.19). The driest part of the Prairies is the south-eastern area of Saskatchewan and the south-western portion of Alberta; soil moisture increases as we move north from this area, and the colour of the Ah horizon grows darker as the amount of OM stored increases in response to the moister conditions. Often the Ah and the Bm horizon show evidence of the activity of burrowing animals, which is recognized with the u suffix (e.g., Ahu, Bmu). Chernozemic soils also typically have a well-developed C horizon with secondary calcium carbonate (Cca horizon), which overlies a C horizon with the original calcium carbonate inherited from the parent material (Ck horizon).

The climatically controlled increases in OM storage (and surface soil colour) leads to a clear zonation of soils that is reflected in the great groups of the Chernozemic order (Brown, Dark Brown, Black, and Dark Gray) (Figure 8.20). The northernmost extent of the Chernozemic order is in the Peace River Lowlands of Alberta and the boundary between grassland and forest trends southeast through Alberta, Saskatchewan, and Manitoba. The geographic position of the transition is controlled by the dominant movement of the air masses in the region, which break through the Cordilleran barrier in the low elevations west of the Peace River area and then trend southeast as they pass through the region (Pennock et al., 2011).
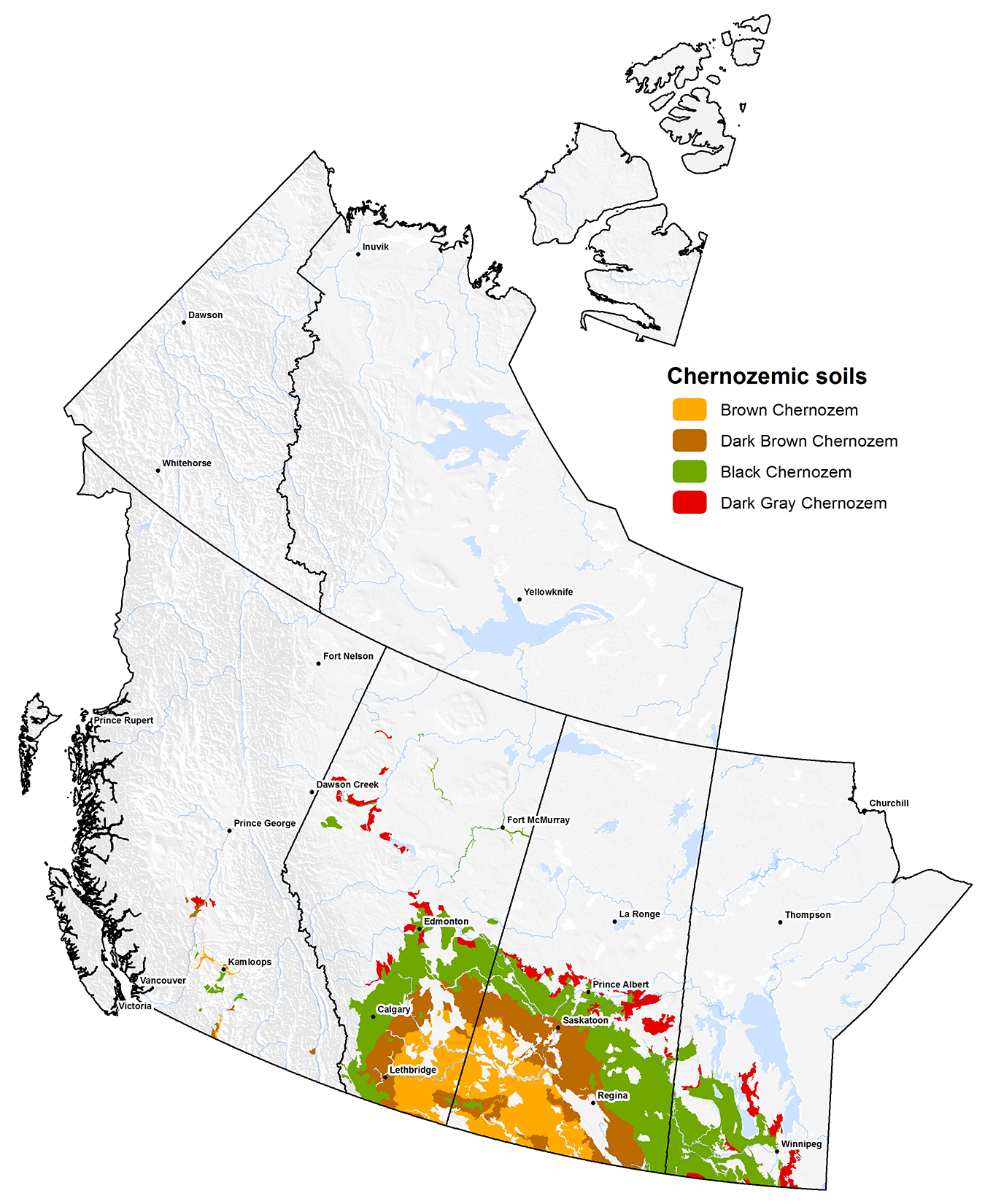
Throughout much of the Prairie region in the transitional zone the Chernozemic soils grade into the Luvisolic soils of the Boreal Plain ecozone. The soils associated with this transitional zone often have A horizons with evidence of both organic additions and bleaching (the Ahe horizon) overlying a weakly developed (or juvenile) texture contrast horizon, designated as a Btj horizon. The soils of the transition are recognized at the great group level as Dark Gray Chernozems or as Dark Gray Luvisols.
The land surface in many areas of the Prairies was formed by melting of immobile ice masses resulting in a hummocky surface form with innumerable small wetlands. A characteristic catena of soils occurs in these small catchments, with Regosolic or thin Chernozemic soils in upper slopes, Chernozemic soils with increasingly thick Ah horizons in mid- and lower-slopes, and Gleysolic soils in the depressions (Figure 8.3).
The other two grassland orders are associated with particular types of parent materials. Vertisolic soils form in clayey glacio-lacustrine parent materials with a high content of smectite clay minerals (Brierley et al., 2011). This causes the soil to shrink in dry periods and form deep cracks that can extend a meter or more into the soil (Anderson, 2010). In wetter periods the clays swell and close the cracks and generally cause mixing or turbation of the soil material. The mixing prevents well-expressed horizons from forming. The turbated horizons are denoted with a v suffix (e.g., Bv, Cv). The displacement of soil material along the cracks also forms polished surfaces (called slickensides) when one soil mass slips by another during turbation. These features are assigned a ss label (e.g. Bss). The presence of horizons with both the v and ss suffixes are required for classification into the Vertisolic order (Figure 8.21).

High concentrations of the base cation sodium (Na+) are responsible for the distinctive soil horizons of the Solonetzic order (Miller and Brierley, 2011) (Figure 8.22). Typically for Chernozemic soils the ratio of exchangeable calcium:exchangeable sodium is 30 or greater, but in Solonetzic soils the ratio is below 10. The high sodium levels result from complex interactions between parent material, topography, the groundwater regime, and time (Anderson, 2010b). Horizons with exchangeable Ca:Na ratios less than 10 are assigned an “n” suffix (e.g., Bn). In the field, they often have high clay content and dense, impermeable structure (often informally called hardpan) and are additionally assigned a “t” suffix (Bnt). The ponding of water on the surface of the Bnt can lead to destruction of the upper B horizon and formation of an Ae/AB layer.

Probably the soil property of greatest interest to producers is salinity but the CSSC does a poor job of recognizing salinity in its taxonomic hierarchy. Horizons with salts inherited from the parent material are assigned an s suffix (e.g., Csk) and those with secondary salts formed through precipitation of ions from groundwater are assigned an sa suffix (e.g., Asa, Bsa). Soils with these horizons are not, however, recognized at the order, great group, or subgroup level of the CSSC but are designated as a phase – for example, Orthic Brown Chernozem, saline phase.
HUMAN IMPACT ON SOIL DISTRIBUTION
Regosols and Anthroposols
The most widespread impact of human activity on soil horizonation (and hence classification) is due to human-induced increases in soil erosion, especially erosion caused by tillage in agricultural landscapes (FAO, 2019). Tillage causes mixing of the surface layer to the depth of tillage (typically between 10 to 25 cm). The tilled surface layer is denoted with a p (ploughed) suffix (Ap). As erosion removes surface soil, ploughing incorporates B and C horizons into the Ap horizon, which can limit plant growth where a growth-limiting B or C horizon exists. Often tillage erosion and mixing eliminates the B horizon totally, and the Ap/C or Ap/Cca sequence is classified into the Regosolic soil order (Figure 8.23). Soil catenas in agricultural landscapes commonly include Regosolic soils in the most eroded positions. Deposition of soil at the base of the slope causes overthickening of the A horizon in the depositional positions but typically this has little effect on the classification of the soil.

A second type of widespread human impact on soils is caused by removal, storage and replacement of soil material associated with various forms of resource exploitation – for example, trenching for pipelines or removal of surface material to allow mining of bitumen in the oilsands or mineral resources elsewhere. The highly disturbed or reconstructed soils can not be adequately described using the terminology of the CSSC, and Naeth et al. (2011) suggested that a new order called the Anthroposols should be adopted to describe them. The diagnostic horizon of the Anthroposols would be the D (for disturbed) horizon. Many other national and international systems of soil classification have already adopted orders to account for these soils. The Anthroposol order has not been officially adopted into the CSSC at the time of writing (summer of 2019).
GLOBAL SOILS
Because of the relative youthfulness of Canadian soils and the limited range of climates found here there are many classes of global soils that do not occur in Canada. The CSSC is designed only for soils found in Canada and we have to use other classification systems for global soils that do not have Canadian equivalents.
Unfortunately, there is no single agreed-upon international soil classification system in place. The two main international systems are Soil Taxonomy (developed by the United States Department of Agriculture) and the World Reference Base for Soil Resources, which was originally developed by the Food and Agriculture Organization of the UN and is now edited by a working group of the International Union of Soil Sciences. The situation is further complicated because the highest taxonomic levels in each system do not correspond very closely to orders in the other system, but despite this overlap in classes a general table of concordance between the two systems (and with the CSSC where appropriate) can be developed (Table 8.3). For our purposes (at the introductory level) we will only use the USDA soil classes as many sources used by Canadian students use the USDA classification. Overall, the lack of a single taxonomic system for soils is a very unsatisfactory state of affairs, leading to significant confusion among generations of students and among scientists and policymakers who wish to use soil information in their work.
Table 8.3. Correlation between classes of Soil Taxonomy, the World Reference Base for Soil Resources, and the Canadian System of Soil Classification
Canadian System of Soil Classification | World Reference Base | Soil Taxonomy1 | |
---|---|---|---|
Order | Reference Soil Group | Order2 | |
Soils with strong human influence | |||
Altered by long human use | Anthrosol | ||
Contains significant human artifacts | Technosol | ||
Permafrost Soils | |||
Permafrost-affected | Cryosol | Cryosol | Gelisol |
Grassland soils | |||
Clay-enriched subsoil, high sodium concentration | Solonetzic | Solonetz | Natric great groups |
High shrink-swell clays, slickensides | Vertisol | Vertisol | Vertisol |
High soluble salt concentration | Solonchak | Salids | |
Dark, well-structured Ah, secondary CaCO3 | Chernozemic (Black GG) | Chernozemic | Mollisol |
(Udolls) | |||
Dark Ah, secondary CaCO3 | Chernozemic | Kastanozem | Mollisol |
(Dark Brown, Brown GG) | (Ustolls, Xerolls) | ||
Dark Ah, no CaCO3 | Phaeozem | Mollisol | |
(Udolls, Albolls) | |||
Dark Ah, low base status | Brunisol | Umbrisol | |
(Sombric) | |||
Arid Zone Soils | |||
Accumulation and cementation by silica | Durisol | Aridisol | |
Durids | |||
Accumulation of secondary gypsum | Gypsisol | Aridisol | |
Gypsids | |||
Accumulation of secondary carbonates | Calcisol | Aridisol | |
Calcids | |||
Wetland Soils | |||
Thick organic Layer | Organic | Histosol | Histosols |
Mineral soil, gleyed properties | Gleysolic | Gleysol | Aquic suborders |
Stagnant water, abrupt textural difference | Planosol | Albaqualfs, | |
Albaquults, | |||
Argiabolls | |||
Stagnant water, no textural contrast | Stagnosol | ||
Forest soils, younger soil surfaces | |||
Illuviation of iron and aluminum | Podzolic | Podzol | Spodosol |
Higher-clay B horizon | Luvisolic | Luvisol | Alfisols (High-activity clays) |
Reddening of B horizon | Brunisolic | Cambisol | Inceptisol |
Soils of older soil surfaces | |||
Illuviation and accumulation of Fe, irreversible hardening when dry | Plinthosol | Plinth- | |
descriptor | |||
Low-activity clays, high Fe oxides | Nitisol | Alfisols, Ultisols (Kandic GG) | |
Dominance of kaolinite and oxides | Ferrasol | Oxisol | |
Interfingering of coarse material into redder layer | Retisol | Gloss- | |
descriptor | |||
Clay-enriched subsoil, low-activity clays, low base status | Acrisol | Ultisols (with low-activity clays | |
Clay-enriched subsoil, low-activity clays, high base status | Lixisol | Alfisols (with Low-activity clays) | |
Clay-enriched subsoil, high-activity clays, low base status | Alisol | Ultisols (with high activity clays) | |
Soils with Little or No Profile Differentiation | |||
Very sandy soils | Arenosol | Psamments | |
Stratified fluvial,lacustrine, or marine | Regosol | Fluvisol | Fluvents |
sediments | (Cumulic) | ||
No significant profile development | Regosol | Regosol | Entisols |
Thin soil or with many coarse fragments | Leptosol | Entisols | |
Soils in volcanic sediments, complex aluminum minerals | Andosols | Andisols | |
1Correlation between USDA and WRB from IUSS Working Group WRB. 2015. World Reference Base for Soil Resources 2014, update 2015. International soil classification system for naming soils and creating legends for soil maps. World Soil Resources Reports No. 106. FAO, Rome. (http://www.fao.org/3/i3794en/I3794en.pdf) 2Soils that correlate at lower taxonomic levels in Soil Taxonomy are shown in italics. |
The additional classes in the USDA/WRB systems (compared to the CSSC) are required (in part) because of the higher degree of chemical weathering in many global soils compared to the relatively youthful, post-glacial soils in Canada. Both the WRB and USDA systems use two criteria as indicators of the degree of weathering. The first, is the base status of the soil – high base status (i.e., the soil exchange complex is dominated by calcium, magnesium, potassium and sodium) indicates limited weathering and leaching, and low base status (i.e., replacement of the four bases by aluminum and hydrogen) indicates greater weathering and leaching. The second, is the exchange capacity or activity of the clay minerals present – complex, inherited clay minerals such as illite or smectite are high activity clays whereas simpler, secondary clay minerals such as kaolinite are low activity. Formation of low activity clays and oxides is associated with older, highly weathered landscapes.
In Canada, the major forest soil on neutral-alkaline parent materials is the Luvisol. On older, non-glaciated surfaces the clay minerals in the Luvisols undergo further weathering and the complex, inherited clays are gradually transformed into low-activity clay variants of the Alfisol order (Figure 8.24). In the next phase of soil formation, chemical weathering further transforms the primary, inherited minerals into secondary iron and aluminum oxides and soils with very-well developed texture contrast horizons and high oxide levels occur. These are classified into the Ultisol order in Soil Taxonomy (Figure 8.24). Finally, the inherited clay minerals are weathered completely and the soil becomes dominated by the simple clay mineral kaolinite and clay-sized iron and aluminum oxides. These soils also often experience very high levels of turbation by soil-dwelling organisms such as ants and termites. Overall the soils are often very thick and have relatively limited horizon development. These soils are classified as Oxisols in the USDA (Figure 8.24). In some situations a horizon with very high oxide levels forms in the soil, which is called plinthite; when exposed to the atmosphere this material can irreversibly dry, creating rock-like petroplinthite. These soils are informally referred to as laterites.
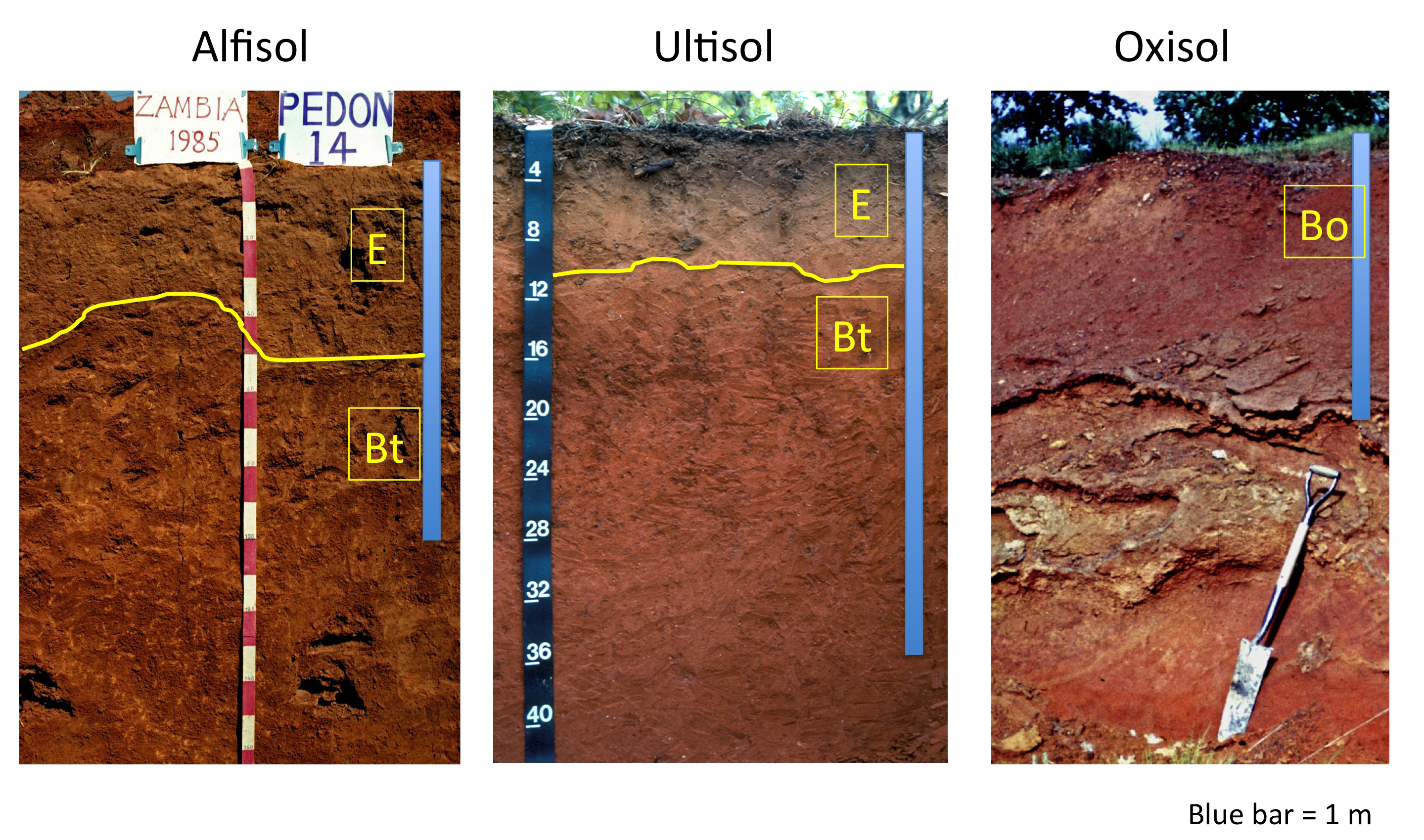
Ultisols are the dominant soil order in the Southwestern USA and throughout much of south-east Asia (Figure 8.25). They also occur fringing the soils of the Oxisol order in the older land surfaces in South America and equatorial Africa. Many of the Alfisols found in South America and the Sahel region of Africa would be of the low-activity clay variants. Paton et al. (1996) argue that soil classification systems such as the USDA Soil Taxonomy are inadequate for countries like Australia, which generally has old land surfaces with complex environmental histories.
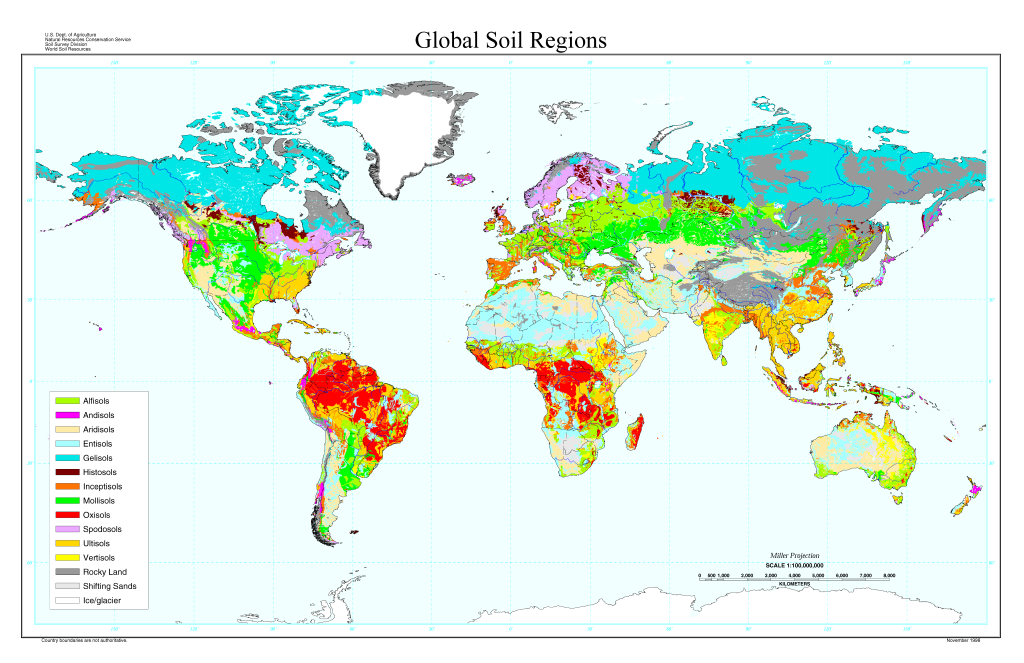
This brief summary necessarily simplifies the great complexity of soil formation on older, highly weathered land surfaces, many of which have undergone substantial changes in environmental conditions over time (Paton et al., 1996). It does, however, highlight a major difference between Canadian soils and these more heavily weathered soils: many Canadian soils have high levels of primary (i.e., inherited from the parent material) minerals present which release a steady supply of mineral nutrients (such as calcium, magnesium and potassium) for plant growth through time. For agricultural soils in the Canadian Prairies (as an example), it is relatively rare to add potassium from processed potash rock, but many more heavily weathered soils require potassium fertilizer additions to achieve optimum crop yields.
True hot desert soils do not occur in Canada. Desert soils in Soil Taxonomy are grouped into the Aridisols order. Desert soils with cemented layers of silica, gypsum, and calcium carbonate are recognized at lower taxonomic levels in the USDA system (Table 8.3). Many desert areas also have thin, weakly developed soils of the Entisol order, comparable to Regosolic soils in the CSSC.
Both the USDA and WRB systems also have classes for soils formed in volcanic sediments, primarily volcanic ash. These soils have a distinct suite of properties and are classified in the Andisol order. They occur in small areas associated with active volcanic areas in plate boundary areas (e.g., Japan, southern Chile).
SOIL SURVEYS
The primary reason for developing soil classification systems is to allow soils to be consistently mapped and the histories of soil classification and soil mapping are inextricably intertwined. Both began in the early 20th century as countries began to systematically inventory their natural resources. Soil mapping has always been referred to as soil surveying, and the maps themselves are referred to as soil surveys.
In Canada, soil surveys began in Ontario in 1914 followed by British Columbia and the Prairie provinces in the 1920s, Quebec and the Atlantic Provinces in the 1930s, and finally the Yukon, Northwest territory and Nunavit in the 1940s and 1950s (Anderson and Smith, 2011; SCWG, 1998, Chapter 1). Federal government involvement in soil surveys essentially ceased across Canada in the early 1990s, but some provinces have continued surveys since that time.
Soil survey therefore began before a national classification was in place—the initial version of the CSSC was published in 1970. Subsequent editions of the CSSC were published in 1987 (2nd Ed.) and in 1998 (3rd Ed.). This can be a source of confusion to users who consult paper surveys published through the main era of surveys (from 1945 to the early 1990s) as the classification used may be archaic and not readily translated to the 1998 edition of the CSSC. Updating of the classification systems has occurred in the on-line versions of the soil surveys (as discussed below).
There have been a wide variety of scales used for the soil surveys in different provinces. The scale at which a survey is carried out largely determines its usefulness for land use planning and resource assessment – generally the smaller the scale, the more limited the map is for planning purposes. Generally, soil surveys in British Columbia, Manitoba, Ontario, Quebec, Nova Scotia, New Brunswick, P.E.I., and Newfoundland were produced at scales between 1:20,000 and 1:63,360 (i.e., Survey Intensity Levels 2 and 3, Table 8.4). Surveys in Alberta were a mix of scales between 1:50,000 and 1:126,730 and surveys in Saskatchewan, the Yukon and Northwest Territories, and Nunavut are at scales of 1:100,000 or greater. Information on all surveys is available on-line at http://sis.agr.gc.ca/cansis/publications/surveys/index.html.
The basic process used in field work for soil surveys is similar across Canada (although each province has its own variant of the basic process). First, a Survey Intensity Level (SIL) is chosen based on the purpose of the survey (Table 8.4). The SIL determines the number of soil profiles (inspections) that will be described per unit area. Normally, the soil surveyor chooses an inspection point that they believe is most representative of the area being described. At each profile (using soil pits or soil exposures at roadsides, trenches etc.) the soil profile is divided into horizons and a standard suite of soil properties are described. Each horizon is assigned labels according to the CSSC and these labelled horizons are used to classify into the correct order, great group, and subgroup level of the CSSC. The geographic position, landform context of the point is recorded, and the nature of the parent material is also recorded.
The soil surveyor must then delineate the area of land that they believe the soil they have described is representative of. As we have seen in Chapter 2, in Canada the parent material is of primary importance in determining the characteristics of the soil and hence the described soil pit is (hopefully) representative of the parent material it occurs in. The extent of the parent material was normally mapped using aerial photographs – different types of parent material often have characteristic surface forms associated with them (Tables 2.1 and 2.2).
The combination of a given soil class and its parent material is called the soil series, which is the basic unit for the construction of soil maps across Canada (although it is confusingly referred to by different names in different provinces). By convention across Canada series are assigned geographic names based on the closest locality to the point at which they are first described. This means that the names for series are specific to each province, although by referencing the soil taxonomic class and parent material, correlations across provinces are readily done. In some provinces, the soil phase is used to further subdivide soil series – soil drainage class is a commonly used phase criteria in much of Central and Atlantic Canada for example.
For soil maps beyond the most intensive SIL 1 level it is not possible to show individual soil series on the traditional paper map. Instead map units that contain multiple series are used. Often these map units can be related to soil catenas as described earlier in this chapter (although this differs by province). In a simple map unit one series dominates, but in complex units two or more series may occur. In this case the label for each mapped area (called map polygons) will often give an estimate of the percentage of each series in the polygon.
As the scale of survey increases, the number of series in each map polygon will normally also increase and hence the variability in soils in each polygon becomes greater. This variation limits the usefulness of the map for specific planning purposes and hence the broadest surveys at reconnaissance through exploratory levels (Table 8.4) are only of use for very generalized planning purposes.
Table 8.4. Usual scale, inspection intensity, and general objective of the survey intensity levels used in soil surveys in Canada. From Agriculture and Agri-Food Canada (1987).
Survey Intensity Level (SIL) and Name | Usual scale | Inspection (soil pit) Intensity | Objective |
---|---|---|---|
SIL 1 Very detailed |
1:5,000 (1 cm on map equals 50 m on ground surface) |
One per 1 to 5 ha | Very intensive planning e.g. for high value crops (nurseries), septic fields, or baseline and post-disturbance pipeline surveys |
SIL 2 Detailed |
1:20,000 (1 cm on map equals 200 m on ground surface) |
One per 2 to 20 ha | Local planning for groups of farms, catchments, small parks, irrigation management |
SIL 3 Reconnaissance |
1:50,000 (1 cm on map equals 500 m on ground surface) |
One per 20-200 ha | Limited number of uses for county planning, large parks, major stream catchments, irrigation districts |
SIL 4 Broad Reconnaissance |
1:100,000 (1 cm on map equals 1,000 m (1 km) on ground surface) |
One per 100-1000 ha | Limited number of uses: provincial level suitability assessments, regional plans |
SIL 5 Exploratory |
1:250,000 (1 cm on map equals2,500 m (2.5 km) on ground surface) |
Greater than 1 per 1000 ha; often widely separated | Very limited general uses: broad regional or provincial plans |
The province-specific approaches to mapping make it very difficult to develop a Canada-wide soil map based on the provincial surveys. To address this problem Agriculture and Agri-Food Canada produced the Soil Landscapes of Canada map at a scale of 1:1,000,000 (http://sis.agr.gc.ca/cansis/nsdb/slc/index.html). This map is the only comprehensive soil map of Canada. Each polygon on the SLC map describes one or more particular type of soil and the landscape associated with it. Version 3.2 of the SLC map was released in March 2011 and is the basis for the maps (Figure 8.1) used in this textbook.
CANADA LAND INVENTORY
The Canada Land Inventory (CLI) was a major effort by a number of federal government agencies to map and classify natural resources in Canada. The CLI was established by the Agricultural Rehabilitation and Development Act of 1961 and mapping began in 1963 and was essentially complete in 1975-76. The CLI covered approximately 2.6 million km2 (or about 25% of the Canadian land mass) and included assessment for agriculture, forestry, outdoor recreation, and wildlife (Pierce and Ward, 2013). The capability of mapped areas for each activity was placed into 7 classes, with class 1 being the highest capability and class 7 the lowest. Classes 1 to 3 were deemed to be the prime lands for each activity.
The determination of CLI class is made by first assessing the limitations present in each area and severity of the limitation. The area of land being assessed was then assigned the value of the most severe limitation present to arrive at its final class (i.e., from 1 to 7).
Information on soils was of greatest relevance for the agricultural and forestry CLI classes. The agricultural CLI classes required information on ten main criteria: undesirable soil structure and/or low permeability, erosion damage, low natural fertility, inundation risk, low moisture holding ability, surface stoniness, adverse climate (heat units available for growth and/or moisture), salinity, shallow bedrock, and adverse topography. Information on the human context of agriculture (e.g., distance to markets, road infrastructure) was not considered. Note that the soil taxonomic class was not part of the CLI system – this lack of use of the soil taxonomic system in assessments like the CLI was one factor that limited the use of soil surveys by the broader community and contributed to the withdrawal of federal funding for surveys in the 1990s.
The forestry CLI classification included the soil factors used in the agricultural system. It also included factors relating to soil moisture (soil moisture deficiency, soil moisture excess), permeability and rooting depth (physical restriction to rooting caused by dense soil horizons or shallow bedrock) as well as various climate factors.
CLI classes are still used in some jurisdictions in Canada for land use planning purposes. In Ontario, for example, the 2014 Provincial Policy Statement under the Planning Act requires that prime agricultural areas (i.e., those in CLI classes 1 to 3) must be protected and designated for long-term agricultural use (OMAFRA, 2019b).
Can You Dig It!
“Oh, give me land, lots of land under starry skies above….”
How can we compare different provinces and countries in terms of their natural endowment of agricultural land? The most used measure is the area of arable land per person (measured in hectares per person). In Canada we can use the Canada Land Inventory to readily compare across provinces. The highest is Saskatchewan, with an amazing 14.67 ha per person; the lowest is Quebec with only 0.28 ha per person, followed closely by BC and Ontario. However even Quebec has higher values than the global average – according to the World Bank, in 2016 the global average was only 0.19 ha per person. This average has declined from an average of 0.37 ha per person in 1961. Only a few countries have a greater natural endowment than Canada (which has an average of 1.21 ha per person according to the World Bank). For major countries only Kazakhstan (1.65 ha/person) and Australia (a whopping 1.90 ha/person) outstrip Canada.
Soil resources in Canada (Canada Land Inventory Capability Classes 1, 2 and 3*‡) compared with population (estimated for 2019). Table compiled by Tim Moore, McGill University.
Region | CLI 1 | CLI 2 | CLI 3 | ΣCLI 1-3 | Population | ΣCLI 1-3 /population |
---|---|---|---|---|---|---|
(thousands of km2) | (million) | (ha per person) | ||||
Atlantic | 0 | 5.89 | 22.78 | 28.67 | 2.4 | 1.19 |
QC | 0.6 | 9.54 | 13.63 | 23.77 | 8.4 | 0.28 |
ON | 22.49 | 23.6 | 32.79 | 78.87 | 14.4 | 0.55 |
MB | 1.83 | 25.56 | 25.61 | 53.01 | 1.4 | 3.79 |
SK | 10.72 | 64.46 | 100.82 | 176.00 | 1.2 | 14.67 |
AB | 7.08 | 38.97 | 62.88 | 108.93 | 4.3 | 2.53 |
BC | 0.7 | 3.98 | 10 | 14.68 | 5.0 | 0.29 |
*From the Canada Land Inventory https://sis.agr.gc.ca/cansis/publications/maps/cli/1m/agr/index.html ‡For descriptions of the Land Capability Classes, see https://sis.agr.gc.ca/cansis/nsdb/cli/classdesc.html |
DIGITAL SOIL MAPPING AND THE FUTURE OF
SOIL SURVEY
In many areas of the world including parts of Canada, traditional soil survey has been replaced with computer-assisted approaches that are generally termed Digital Soil Mapping (DSM). DSM draws upon all the resource information (including existing soil maps and soil profile data) available for a given location to predict the likely range of soil properties and soil taxonomic classes present at that point. The predictions are especially accurate where high-resolution topographic information is available such as that produced by laser light surveying (i.e., light detection and ranging, or LIDAR). The algorithms that produce the predictions and maps can also estimate the likely error associated with the predictions and hence allow users to assess the uncertainty associated with the maps. The techniques associated with DSM are discussed in more detail in Chapter 17.
SUMMARY
- Soils in Canada are classified according to the Canadian System of Soil Classification (CSSC), a formal taxonomic system with a hierarchy of six levels.
- The highest level in the CSSC, the order, is based on diagnostic horizons that reflect the effects of the dominant soil-forming process. There are 10 soil orders. The next level, the great groups, are based on differences in the strength of the dominant process or contribution of an additional soil-forming process.
- The distribution of soils at different scales (e.g., hillslope, catchment, region, province) is controlled by seven soil-forming factors: climate, organisms, parent material, topography, time, groundwater, and human activity.
- Different classes of forest soils occur on the two main parent materials types in Canada. The CSSC distinguishes between forest soils formed in acidic parent materials (pH levels less than 5.5) and those formed in neutral-alkaline parent materials (pH levels of 5.5 or greater).
- Forest soils formed in acidic parent materials are classed into the Podzolic order and the Brunisolic order depending on the amount of iron, aluminum, and OM deposited in the middle or B horizon of the soil.
- Forest soils formed in neutral-alkaline parent materials are classified into the Brunisolic soils and into the Luvisolic order depending on the differences in clay content between the B horizon and the mineral horizon that overlies it.
- The presence of permafrost layers is diagnostic for soils of the Cryosolic order and the presence and absence of frost mixing is recognized at the great group level.
- The organically enriched surface soil horizon of grassland soils is diagnostic of the Chernozemic order. Grassland soils on clay-rich parent materials with mixing of horizons due to expanding clays are classified into the Vertisolic order, and those with high sodium contents into the Solonetic order.
- Wetland soils with thick (> 40 cm) mats of peat are classified into the Organic order, and mineral wetland soils that have gley features such as dull colors and mottling are classified into the Gleysolic order.
- The scope of the CSSC is limited to those soils found in Canada. For soils not found in Canada either the American USDA system (Soil Taxonomy) or the United Nations system (World Reference Base) is used. The existence of multiple classification systems is a weakness of soil science as a discipline.
- The soils of Canada have been surveyed (or mapped) at various scales since the beginning of the 20th century. The usefulness of a given soil survey for land use planning is determined by the scale of the survey.
- Soils data were extensively used in the Canada Land Inventory, which was a major effort in the 1960s and 1970s to map the capability of activities such as agriculture and forestry. The results of the inventory are still used for land use planning in some jurisdictions in Canada.
SUGGESTED READING
Soil Classification and Description for Canada:
Images for Canadian soils and additional soil features required for classification can be found in the Imagery Collection of the Soils of Canada website
Information on soil orders and soil classification keys are in the Field Handbook for the Soils of Western Canada. Available at https://soilsofcanada.ca/links.php
USDA Soil taxonomy: Good summary of the properties of each order in soil taxonomy is available from the University of Idaho at https://www.uidaho.edu/cals/soil-orders
In addition to the Field Handbook, there are two books that are very useful in field description of soils:
Heck, R., Kroestch, D.J., Lee, H.T., Leadbetter, D.A., Wilson, E.A. and Winstone, B.C. 2017. Characterizing Sites, Soils & Substrates in Ontario – Volume 1 Field Description Manual. School of Environmental Sciences, University of Guelph. Information about this book is available at:
https://www.uoguelph.ca/ses/sites/default/files/CSSSO%20Vol1%20Announcement.pdf
Watson, Kent. 2007 (Revised 2009, 2014). Soils Illustrated. International Remote Sensing Surveys Ltd., Kamloops, B.C. Information on this book is available at:
STUDY QUESTIONS
- What are the seven factors of soil formation? Which factor do you think is most responsible for
the sequence of soils seen in Figure 8.3? - Which three diagnostic horizons result from the addition of organic material? What setting (e.g.,
forest, tundra, wetland etc.) would each one likely to be found in? - Which two diagnostic horizons result from turbation of soil material? What factor of soil
formation do you think is most responsible for each horizon? - In the 1930s parts of the Prairie Provinces experienced very severe wind erosion. What soil
would have been associated with the wind-eroded surfaces? What factor of soil formation do
you think was most responsible for these soils? - Salts are associated with very dry soils yet their distribution in the landscape is largely
determined by water. Briefly resolve this conundrum. - What is key threshold used by the Canadian System of Soil Classification (CSSC) to distinguish
between the two main types of parent materials for forest soils? - The great Canadian pedologist Paul Sanborn has argued that Folisols are the hardest soils to dig
a soil pit in. Based on Figure 8.12 why do you think that this is the case? - What two soil forming factors are most responsible for the soil zones (Figure 8.20) of the
Canadian Prairies? - What two criteria of weathering are used by Soil Taxonomy and the World Reference Base
systems of soil classification? - You have been asked to find a route for a pipeline using a soil survey completed at Survey
Intensity Level 4. Is this feasible? Briefly explain your answer.
REFERENCES
Agriculture Canada. Research Branch. 1987. Soil survey handbook. Volume 1. Technical Bulletin 1987-9E. Available at: http://sis.agr.gc.ca/cansis/publications/manuals/1987-9/1987-9-soil-survey-handbook.pdf
Anderson, D.W. 2010. Vertisolic soils of the Prairie Region. Prairie Soils and Crops Journal 3: 29-36. Available at: https://prairiesoilsandcrops.ca/volume3.php
Anderson, D.W. and Cerkowniak, D. 2010. Soil formation in the Canadian Prairie region. Prairie Soils and Crops Journal 3: 57-64. Available at: https://prairiesoilsandcrops.ca/volume3.php
Anderson, D.W. and Smith, C.A.S. 2011. A history of soil classification and soil survey in Canada: Personal perspectives. Can. J. Soil Sci. 91: 675-694.
Bedard-Haughn, A. 2011. Gleysolic soils of Canada: Genesis, distribution and classification. Can. J. Soil Sci. 91: 763-779.
Brierly, J.A., Stonehouse, H.B., and Mermut, A.R., 2011. Vertisolic soils of Canada: Genesis, distribution and classification. Can. J. Soil Sci. 91: 903-916.
DREE. 1970. The Canada Land Inventory. Objectives, scope and organization. Second Ed., Report No. 1. Ottawa 1970. http://publications.gc.ca/collections/collection_2019/eccc/RE63-1-1970-eng.pdf
Ellis, J.H. 1938. The Soils of Manitoba. Economic Survey Board. Province of Manitoba. September , 1938. 112 pp. Available at: http://sis.agr.gc.ca/cansis/publications/surveys/mb/mbs006/mbs006_report.pdf
FAO. 2019. Soil erosion: the greatest challenge to sustainable soil management. Rome. 100 pp. Available at: http://www.fao.org/3/ca4395en/ca4395en.pdf.
Fox, C.A. and Tarnocai, C. 2011. Organic soils of Canada: Part 2. Upland Organic soils. Can. J. Soil Sci. 91: 823-842.
Jenny, H. 1994. Factors of Soil Formation. A System of Quantitative Pedology. New York: Dover Press (Reprint, with Foreword by R. Amundson of the 1941 McGraw-Hill publication). Download available from NRCS website.
Kroetsch, D.J., Geng, X., Chang, S.X., and Saurette, D.D. 2011. Organic soils of Canada: Part 1. Wetland Organic soils. Can. J. Soil Sci. 91: 807-822.
Lavkulich, L.M. and Arocena, J.M. 2011. Luvisolic soils of Canada: Genesis, distribution and classification. Can. J. Soil Sci. 91: 781-806.
Miller, J.J. and Brierly, J.A. 2011. Solonetzic soils of Canada: Genesis, distribution and classification. Can. J. Soil Sci. 91: 889-902.
Naeth, A., Archibald, H.A., Nemirsky, C.L., Leskiw, L.A., Brierley, A.J., Bock, M.D., VandenBygaart, A.J., and Chansyk, D.S. 2012. Proposed classification for human modified soil in Canada: Anthroposolic order. Can. J. Soil Sci. 92: 7-18.
OMAFRA 2019a. Classifying Prime and Marginal Agricultural Soils and Landscapes: Guidelines for Application of the Canada Land Inventory in Ontario. http://www.omafra.gov.on.ca/english/landuse/classify.htm#groupings
OMAFRA 2019b. FAQ Prime Agricultural Areas. http://www.omafra.gov.on.ca/english/landuse/prime-ag-areas.htm
Paton, T.R., Humphreys, G.S. and Mitchell, P.B. 1996. Soils A New Global View. Yale University Press, New Haven and London. 234 pp..
Pennock, D.J., Bedard-Haughn, A., and Viaud, V. 2011. Chernozemic soils of Canada: Genesis, distribution and classification. Can. J. Soil Sci. 91: 719-747.
Pierce, T.W and N. E. Ward. The Canada Land Inventory. The Canadian Encyclopedia. https://www.thecanadianencyclopedia.ca/en/article/canada-land-inventory.
Sanborn, P., Lamontagne, L., and Hendershot, P. 2011. Podzolic soils of Canada: Genesis, distribution and classification. Can. J. Soil Sci. 91: 843-880.
Smith, C.A.S., Webb, K.T., Kenney, E., Anderson, A., and Kroetsch, D. 2011. Brunisolic soils of Canada: Genesis, distribution and classification. Can. J. Soil Sci. 91: 695-717.
Soil Classification Working Group. 1998. The Canadian System of Soil Classification. Agric. And Agri-Food Canada. Publ. 1646 (Revised). 187 pp. Available at: http://sis.agr.gc.ca/cansis/publications/manuals/1998-cssc-ed3/cssc3_manual.pdf
Tarnocai, C. and Bockheim, J.G. 2011. Cryosolic soils of Canada: Genesis, distribution and classification. Can. J. Soil Sci. 91: 749-762.
VandenBygaart, A.J. 2011. Regosolic soils of Canada: Genesis, distribution and classification. Can. J. Soil Sci. 91: 881-887.
About the Author
Daniel J. Pennock, Professor Emeritus, Department of Soil Science, University of Saskatchewan

Dan Pennock is a Professor Emeritus (or, more simply, a retired professor) of the Department of Soil Science at the University of Saskatchewan. His research career focused on how soils are shaped by their place in the landscape and by the processes (like erosion or water movement) that occur in landscapes. He taught courses in many areas of soil science and geography, and his love of teaching was recognized by the university with a Master Teacher award in 2006. He was made a Fellow of the Canadian Society of Soil Science in 2010. Since his retirement he has collaborated on a number of projects on sustainable management of soils with the Global Soil Partnership of the Food and Agriculture Organization of the United Nations.
It is the smallest, three-dimensional unit at the surface of the earth that is considered as a soil. Its lateral dimensions are 1 m if ordered variation in genetic horizons can be sampled within that distance or if these horizons are few and faintly expressed.
The interrelated natural agencies responsible for the formation of soil. The factors are typically grouped into following five categories: parent material, climate (precipitation, temperature), organisms (flora and fauna), topography (elevation, slope, depth to water table), and time.
A group of soils that commonly occur together in a landscape, each characterized by a different slop position and resulting set of drainage-related properties.
The extent to which the adsorption complex of a soil is saturated with exchangeable cations other than hydrogen and aluminum; expressed as a percentage of the total cation exchange capacity.
Polished and striated surfaces that occur along planes of weakness resulting from the movement of one mass of soil against another in soils dominated by swelling clays; commonly observed in Vertisols.
A comprehensive multi-disciplinary land inventory of rural Canada, covering over 2.5 million square kilometers of land and water.Includes land capability for agriculture, forestry, wildlife, recreation, wildlife (ungulates and waterfowl).
There are seven classes used to rate agricultural land capability, with Class 1 lands having the highest and Class 7 lands the lowest capability to support agricultural land use activities.